The Higgs boson is one more particle we know how to identify now, so we can now focus on new exotic phenomena that might produce Higgs bosons in the final state, and entertain ourselves in their search.
In addition to having one more particle to play with, there is the possibility that the 125 GeV Higgs boson discovered in 2012 is only one of a multiplet of new particles, all originated through the same mechanism called "electroweak symmetry breaking" (EWSB), which first brought theorists to hypothesize the existence of the Higgs particle. After all, while there is only one Higgs particle in the simplest instantiation of the EWSB mechanism, one just needs to make the theory an inch more complex to come up with four additional Higgs-like particles.
Such extensions of the "minimal" Standard Model are called "two-higgs-doublet-models", 2HDM for insiders. Among the wide class of 2DHM are the simplest instantiations of Supersymmetry; yet finding new Higgs bosons would not automatically prove that the world is Supersymmetric. It could just be that Nature (the bitch, not the magazine) has chosen that two (doublets) are better than one.
The two possibilities pointed out above - using the 125 GeV Higgs as a final state signal of something else, and searching for new Higgs particles - have been exploited together in a recent search performed by the CMS collaboration. One of the five Higgs bosons predicted in 2DHMs is called the "A" boson - it is a boson with slightly different properties from those of the regular Higgs we have found in 2012 (most notably, its "CP" parity, which is negative).
If the A boson is heavier than about 220 GeV, it may decay into a Z boson and a H boson, giving rise to a distinctive final state.
CMS looked in its 8-TeV data sample for decays of the Z to electron-positron or muon pairs, and then in the resulting events looked for two jets identified as originated by bottom quarks. The Higgs boson decays over half of the time in a pair of b-quarks, so that is a profitable way to search for it.
Once events with the "Z+bb" topology are found, one still has to deal with some reducible backgrounds. The most important one is top pair production: if both top quarks decay to a lepton-neutrino-b quark, the final state will include two leptons and two b-quarks, but also a significant amount of missing transverse energy due to the escape of the two energetic neutrinos. Requiring small missing transverse energy thus acts as a practical veto for this background.
Then there is the background from Z production in association with two b-quarks, when the two b-quarks are originated by strong-interaction processes. This is what is called a "irreducible" background, in the sense that it cannot be distinguished by its final state content. Still, the two b-quark jets in these events do not "resonate": their combined mass is not fixed at 125 GeV like in the case of the signal.
To exploit the fact that there is a H->bb decay in signal events, and also a A->ZH decay to start with, a kinematical fit is performed whose aim is to better reconstruct the mass of the particles, in particular the A boson. What the fit does is to "pull" the observed energy of the leptons and jets such that they best fit to the hypothesis that they come from the decay chain A->ZH->llbb. You can pull an experimental measurement by moving the value up and down within its experimental uncertainty; if you pull too much the fit will be poor, and the likelihood that the event is a signal one is low.
The four-body mass distribution is shown in the picture below for signal events generated at different values of the A mass. The distributions before/after corrections show that the fit can recover most of the resolution loss thanks to the constraints present in the event.
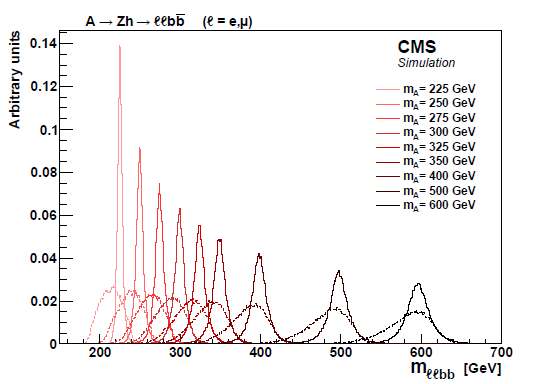
A multivariate discriminant is finally used to separate signal and residual backgrounds. The output of the discriminant is used together with the four-body mass in a two-dimensional fit to signal and background templates. This provides the best sensitivity to the A->ZH process across a wide range of A masses. The projection of the fit on the mass plane is shown in the figure below - there are two more of them because there are three different multivariate discriminants, optimized for different ranges of the mass of the A boson; the one shown is for the "intermediate" mass search.

The red histograms at the bottom in the mass histogram show the expected signal for a 300, 325, and 350 GeV A boson; the black points show the observed data. As shown in the lower insert, which displays the difference between observed data and predicted backgrounds, a small excess is present in this histogram at 310 GeV, not dissimilar to what a signal would look like.
In the end, a limit on the cross section of the A boson is produced as a function of A mass. The limit is shown below. As you can see, there are a couple of mass regions where small excesses in the data bring the observed limit (black curve) outside the yellow band (2-sigma range of expected limits). The global significance of these excesses is however un-exciting.

In summary, a nice new analysis probed a wide region of A mass values, finding no signal. The minimal standard model remains the best guess of what Nature has chosen for us. This search will be repeated with the larger data sample of 13 TeV collisions next year. For the time being, we must congratulate (or better, self-congratulate, in this particular case!) with the authors for a very carefully designed search!

In summary, a nice new analysis probed a wide region of A mass values, finding no signal. The minimal standard model remains the best guess of what Nature has chosen for us. This search will be repeated with the larger data sample of 13 TeV collisions next year. For the time being, we must congratulate (or better, self-congratulate, in this particular case!) with the authors for a very carefully designed search!
Comments