In biology, everything has a history. Creationists love to try to calculate the probability of a new gene spontaneously coming into existence, but that's not how genes are born. New genes most often come from other genes: one gene gets duplicated by a freak accident (like the accidental duplication of a chunk of chromosome, a whole chromosome, or even an entire genome), so that you suddenly have a cell with two working copies of the same gene. As time goes on (that is, time on an evolutionary scale), those two duplicate genes start to divide up the work that was originally done by just one gene. One copy might end up specializing in one particular task, picking up mutations along the way that gradually transform this copy into an independent gene in its own right, with its own specialized function. From one gene, you get two, each with a distinct role in the cell.
It sounds like a nice evolutionary story, but do scientists have any real examples of duplicate genes evolving new functions?
To understand how new genes evolve, you can't do much better than to look at gene evolution in yeast. We have the genome sequences for several dozen yeast species, species that cover a wide range of evolutionary relationships from close relatives separated by only a few million years of evolution (comparable to humans and chimps), to species that last shared a common ancestor hundreds of millions of years ago (comparable to humans and sea squirts). Compared to vertebrates, all that yeast evolution may seem boring - after 400 million years, you still just have a single-celled organism. But on the inside, yeast have undergone tremendous evolutionary change as they've colonized a wide variety of environmental niches, from wine flasks and tree bark to flies and the inside of your gut. And scientists can study all of this evolutionary diversity in the lab, because doing experiments with single-celled yeast is easy. One lab can handle a dozen different yeast species too much hassle. You can't do that with vertebrates- if you want to work with a dozen different vertebrates with the same level of evolutionary diversity, you no longer need a lab - you need a zoo.
Finding Newly Evolved Genes
To find examples of newly evolved genes, scientists can go to the databases and examine the genomes of these dozens of yeast species. The logic is fairly simple: take a species (let's call it species A), and find two genes that bear a strong family resemblance, genes that clearly look like that they were once copies of each other. If these two genes are copies of just one original gene, then you ought to be able to find that one original gene (or more accurately, the present-day descendants of that original gene) in other yeast species. In the evolutionary line leading up to species A, there was a freak accident that produced two copies of one original gene. Since that freak accident didn't happen in the lines leading to species B and C, these species only have one copy of the original gene:
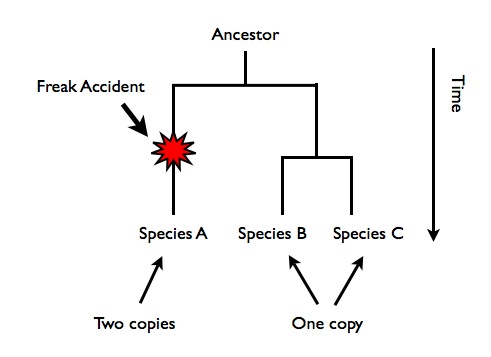
A gene duplication in only one evolutionary line
(Of course, it is a little more complicated than this: it is possible that the ancestor of all three species had two copies of a gene, and one copy was lost in the line leading to species B and Cs. We can eliminate this alternate scenario by looking at more yeast species, but that's another story.)
This common scenario is how many, maybe even most new genes are born. But how do you get from having two identical genes, capable of performing the same functions, to two distinct genes, each with their own role in the cell?
One hypothesis would be that the original gene, in the ancestor species, had multiple jobs to do, and after the freak accident the two copies split the work load, each one becoming specialized at a different task. If this hypothesis is true, then you can make a prediction about what we should find in today's living species: in species A, the two related genes will now do different specialized jobs. In species B and C, where you have just one uncopied gene, that gene should be doing both jobs.
This is a nice hypothesis about how genes evolve new functions, but is it right? To know that, you have to go into the lab and do experiments. Here is where yeast really shines in evolutionary studies - it is simple to go into the lab, and see what these different genes are doing in these different yeast species.
Two Genes That Split The Workload
A research group from the University of California in San Francisco has done just that. Writing in PLoS Biology, they describe the function of two genes in baker's yeast (the same yeast you pick up in the baking aisle at Shop 'N Save), called MCM1 and ARG80. These two genes are modified copies of each other. About 100 million years ago, the ancestor of today's baker's yeast, in a major (but actually, not so rare) freak accident duplicated its entire genome, so that every gene had an identical twin. Most of those copies were eventually trashed by mutation, but some gene pairs have persisted. MCM1 and ARG80 make up one of those pairs - they are fairly similar to each other, and more distantly related yeast species only have one copy, MCM1. ARG80 is a gene that was born during the whole genome duplication. The question is, has ARG80 taken over a job that once belonged to MCM1? Is our hypothesis about how genes evolve new functions correct?
MCM1 has many critical jobs it performs in the cell, and without MCM1, cells die. The MCM1 gene codes for a protein called a transcription factor, whose job is switch other genes on and off. MCM1 controls the switch for several types of genes, such as genes that are needed as cells divide, and genes that are needed when yeast get ready to mate.
In many yeast species, MCM1 also controls the genes that are needed to make the amino acid arginine. But in baker's yeast, this job has now been largely assumed by ARG80. The San Francisco group took their yeast into the lab and did the experiments to to show that in two different yeast species without ARG80, MCM1 controls arginine genes, while in baker's yeast, where ARG80 has taken over, MCM1 no longer controls arginine genes (with just a few exceptions). Once the original MCM1 was copied, one copy took over arginine control, and over time became the more specialized gene that we now know as ARG80.
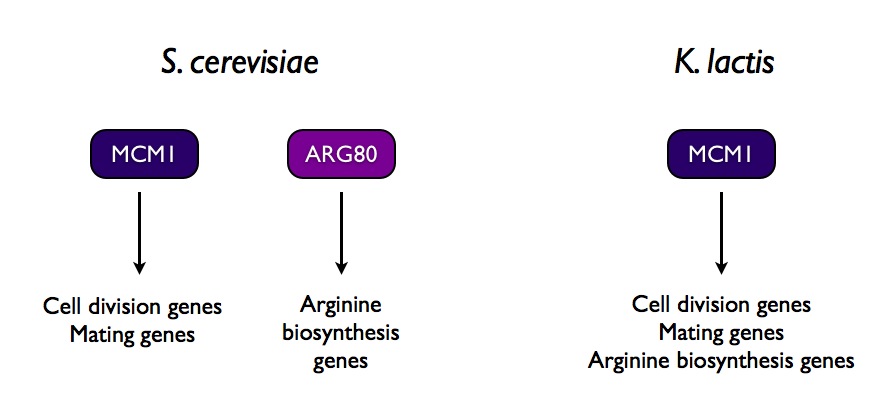
This is a clear-cut example of one way that new genes come into being and take on functional roles in the cell. A gene is accidentally duplicated, and the two copies each take on different jobs, eventually resulting in two distinct genes with two different functions. And those functions aren't just the original functions - MCM1 has picked up new functions in several different species. ARG80 has picked up some new traits too, including the ability to be a better sensor of arginine, which most likely gives baker's yeast more fine-tuned control over arginine metabolism than you could get with MCM1 alone. This is not just an untested evolutionary story, but one which we can verify again and again in the lab with many different examples in yeast. And the examples aren't limited to yeast of course - we also know that newborn human genes often arise by duplication and specialization.
Evolution isn't a science made up of just-so stories. It is a science tested in mant different ways, and most recently, the combination of genome sequences, microbes, and modern molecular biology has let us test evolutionary hypotheses that just a few decades back would have remained interesting, but unverified ideas.
Comments