Atom interferometry, however, hinges on quantum mechanics, the theory that describes how matter behaves at sub-microscopic scales. Just as waves of light can act like photons - particles - atoms can be cajoled into acting like waves if cooled to near absolute zero. At those frigid temperatures, which scientists achieve by firing a laser at the atom, its velocity slows to nearly zero. By firing another series of laser pulses at laser-cooled atoms, scientists put them into what they call a "superposition of states."
The atoms have different momenta permitting them to separate spatially and be manipulated to fly along different trajectories. Eventually, they cross paths and recombine at the detector — just as in a conventional interferometer. "Atoms have a way of being in two places at once, making it analogous to light interferometry," said Stanford University professor Mark Kasevich.
The power of atom interferometry is its precision. If the path an atom takes varies by even a picometer, an atom interferometer would be able to detect the difference. Given its atomic-level precision, "gravitational-wave detection is arguably the most compelling scientific application for this technology in space," said physicist Babak Saif, who is leading the atom optics effort at NASA's Goddard Space Flight Center.
They have designed a powerful, narrowband fiber-optic laser system that it plans to test at one of the world's largest atom interferometers; a 33-foot drop tower in the basement of a Stanford University physics laboratory. Close scientifically to what the team would need to detect theoretical gravitational waves, the technology would be used as the foundation for any atom-based instrument created to fly in space, Saif said.
During the test, the team will insert a cloud of neutral rubidium atoms inside the 33-foot tower. As gravity asserts a pull on the cloud and the atoms begin to fall, the team will use its new laser system to fire pulses of light to cool them. Once in the wave-like state, the atoms will encounter another round of laser pulses that allow them to separate spatially. Their trajectories then can be manipulated so that their paths cross at the detector, creating the interference pattern.
Einstein predicted gravity waves in his general theory of relativity, but to date these ripples in the fabric of space-time have never been observed. Now a scientific research technique called Atomic Interferometry is trying to re-write the canon. In conjunction with researchers at Stanford University, scientists at NASA Goddard are developing a system to measure the faint gravitational vibrations generated by movement of massive objects in the universe. The scientific payoff could be important, helping better clarify key issues in our understanding of cosmology. But application payoff could be substantial, too, with the potential to develop profound advances in fields like geolocation and timekeeping. In this video we examine how the system would work, and the scientific underpinnings of the research effort. Credit: NASA/Goddard Space Flight Center
The team also is fine-tuning a gravitational-wave mission concept it has formulated. Similar to the Laser Interferometer Space Antenna (LISA), the concept calls for three identically equipped spacecraft placed in a triangle-shaped configuration. Unlike LISA, however, the spacecraft would come equipped with atom interferometers and they would orbit much closer to one another — between 500 and 5,000 kilometers apart, compared with LISA's five-million-kilometer separation. Should a gravitational wave roll past, the interferometers would be able to sense the miniscule movement.
"I believe this technology will eventually work in space," Kasevich said. "But it presents a really complicated systems challenge that goes beyond our expertise. We really want to fly in space, but how do you fit this technology onto a satellite? Having something work in space is different than the measurements we take on Earth."
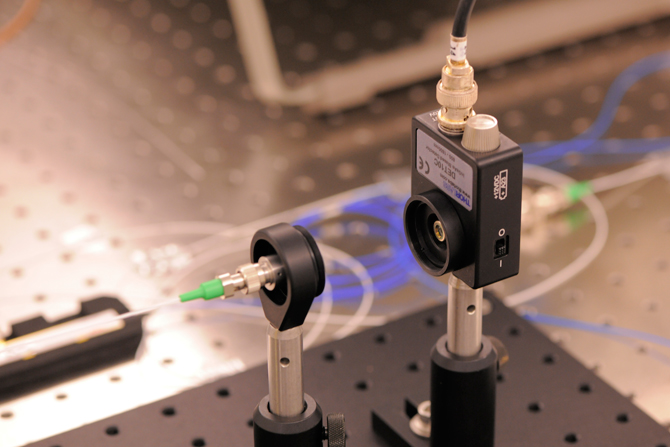
The Goddard-designed breadboard laser system critical to advancing atom-optics instruments. The device will be tested in the Stanford University drop tower. Credit: NASA/Pat Izzo
That's where Goddard comes in, Saif said. "We have experience with everything except the atom part," he said, adding that AOSense already employs a team of more than 30 physicists and engineers focused on building compact, ruggedized atom-optics instruments. "We can do the systems design; we can do the laser. We're spacecraft people. What we shouldn't be doing is reinventing the atomic physics. That's our partners' forte."
Comments