This morning LHC machinists, experimentalists from the LHC experiments, as well as other CERN personnel gathered in the Main Auditorium at CERN to give their end-of-year report. After a LHC status report the spokespersons of ALICE, ATLAS, CMS, LHCB, and TOTEM briefly flashed their first experimental results, obtained from 900 GeV and 2.36 TeV collisions acquired in the course of the last three weeks.
The amount of results that the collaborations have managed to produce in such a small time frame, and from a relatively small amount of collisions, is really astonishing. Of course most of them have no real scientific value, if as a yardstick you use the advancement of our knowledge of particle physics. Yet everybody was eager to know how well the performance of the detectors matches the expectations from computer simulations, and the answer is that all experiments are performing impressively, and that almost everything is understood to a level of detail I myself would have thought improbable to achieve in such a short time frame, just a few weeks ago. But then the first data came, and I saw with my own eyes the quality of the thing we have put together. As a simple starter, have a look at the CMS signal of neutral pions in their decay to photon pairs (a final state of interest for low-mass Higgs boson searches, although at an energy three orders of magnitude larger!). The black points in the histogram below are 900 GeV data, the blue line is the fit including the signal, and the red dashes show the background fit.
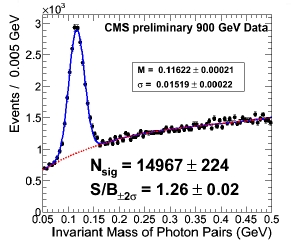
I was especially pleased to see that the effort of the small group of physicists from Padova and Cyprus which my colleague Franco and I have managed to put together has not been vain. We have been working day and night in the last two weeks, in order to produce an approved signal of the

meson. The

is a electrically neutral hadron, composed of a strange-antistrange quark pair. It is a very well-known particle; it was discovered in the fifties and we know everything about it, but it is worthwhile to search its decay: it is a very good calibration line on which to test the detector performance, for a couple of reasons.
Some background on the Phi mesonTo explain those reasons, I need to give you some background. We reconstruct phi meson decays in two charged kaons, as in

. This is the dominant decay mode of the particle -it occurs about 50% of the times- and it is mediated by strong interactions: the strength of the interaction guarantees that it takes place in a time so short that the

has no time to leave the interaction point where it has been created.

Strong interactions are incapable of turning a quark into one of a different species, so the two strange quarks manage to survive inside the kaon daughters. You see that in the sketch on the right (read it from the left to the right, as if time went rightward): strong interactions "fish" a up-antiup-quark pair from the vacuum, and you get two light strange mesons at the price of a heavy hidden-strangeness one. The dashed line is a gluon, which transmits colour charge and momentum to the final state, mediating the decay.
Now, the mass of the

meson equals 1019.4 MeV, which is just a little bit more than twice the mass of the charged kaons (493.7 MeV). With so little extra energy to impart to the daughters, the disintegration pictured above is highly suppressed. This has the consequence that the decay, while "instantaneous", is not as much so as other strong-mediated ones. The

particle has enough time after its production in the proton-proton collision to "resonate" at a well-defined mass: in other words, the strange-antistrange quark pair can reach a well-defined orbit inside the particle, giving it a well-defined mass. So its natural width is unusually small for a strong-decaying hadron, 4.26 MeV.

As for the charged kaons, they live long enough to often cross the entire tracking volume of CMS. They get reconstructed as nice helices in the solenoidal magnetic field, thanks to the small energy they deposit in each of the 13 layers of 300-micron-thick silicon detectors they traverse. Now, it turns out that the energy measured by the silicon layers is a distinguishing feature, which can tell apart different charged particles. You can see it in the figure on the left, which shows how different particles have a different behaviour of deposited energy (on the y axis) which is a unique function of the particle's momentum (the quantity shown on the horizontal axis). The figure is from ALICE (which does not use silicon for this measurement, but the physics is the same), and it is one of the results shown today. I decided to show the ALICE result because in this case it is much better than the CMS or ATLAS plots! ALICE has been designed to excel in the particle identification using the energy release of charged particles, because of its need to study very high-multiplicity events caused by heavy ion collisions.
Reasons why we need to study the phi resonanceSo let us take stock. Here are a few reasons to study the

meson:
1- The

decays without leaving the interaction point, so its decay products constitute a representative sample of so-called "prompt tracks", ones on which to start studying things such as the resolution on the trajectory: since we know where the particles have originated, we have a handle to study the precision of our track reconstruction.
2- The

decays to two kaons: these particles have a quite distinctive energy loss in the silicon, and so

decays allow us to verify in detail and tune our algorithms that exploit that characteristic feature.
3- A pure sample of kaons -which we can select as the two "legs" of the

signal- is also a crucial tool with which to study the characteristics of so-called "decays in flight" of kaons to muon-neutrino pairs. When the charged kaon decays (

), it produces a honest-to-god muon, and we want to use muons as probes of rare, exotic processes, so we have better understand very well how to distinguish these "decay-in-flight" muons from ones produced by the decay of a more exotic object. This is possible because the decay

yields a charged track which has lower momentum than its parent, and the two helices (that of the kaon and that of the muon) may be reconstructed as a single one, but this helix will show odd characteristics, such as large fit chisquared or large impact parameter. So

decays open us a door to a fruitful study of these peculiarities.
4- The

, finally, is a background-ridden signal, not easy to extract from high-energy interactions at the LHC. Finding that particle, and comparing to computer simulations the observed background and signal, is an important step in our learning curve.
The phi signal in early dataNow, I will not spend much time describing the frantic days that have populated the last two weeks of my life. Suffices to say that I have worked about 50% more than what I usually do, or twice as much as I would like to, or three times as much as what I am paid for. A summary of the last week at CERN already exists in this column -I have described it without making reference to the signal I was searching for, because it was not approved material yet.
The Ph.D. student I am co-advising, Luca, can complain further in the comments section if he likes; the same can be said of the undergraduate who also works with us, Pierluigi, and of the post-doc from Cyprus University, Mario. All these folks have lost significant amounts of sleep to put together the single figure I am posting today. Science is tough!
The histogram below shows the mass distribution of opposite-charge pairs of particles reconstructed in the CMS silicon tracker. The tracks have been selected by enforcing the characteristics we expect for kaons produced in

decays: they have helices passing through the interaction point, and have produced energy deposits in the silicon compatible with that expected for kaons. The small bump you observe in the data at about 1019 MeV of mass is due to about a tousand decays of

mesons. The fit returns a reconstructed mass in perfect agreement with book values, and the width is also in great agreement with expectations.
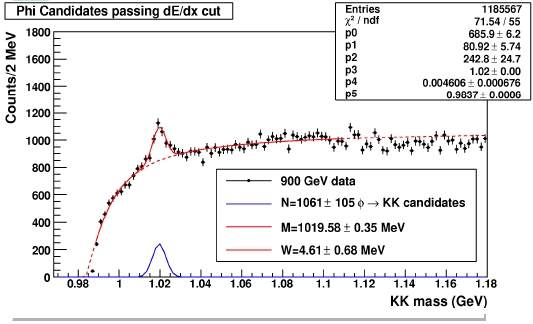
In the next weeks we will use the data collected by CMS so far (about 400 thousand good events at 900 GeV, plus a few tens of thousands at 2.36 TeV) for a preliminary estimate of the probability of kaons, pions, and protons yielding muon tracks, using the

, as well as the

and the

signals shown below. We will also start using the

resonances to study the resolution in the impact parameter of tracks (the impact parameter is the distance of closest approach of the helix of a track from the interaction point) as well as many other characteristics of our reconstructed tracks. It will be exciting!
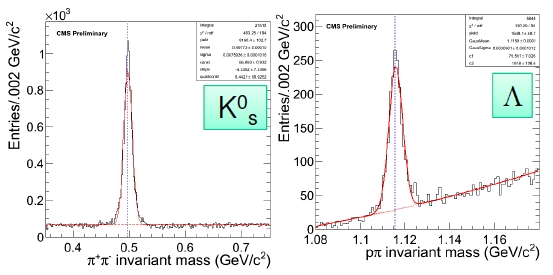
LHC is finally on, and we mean business!
Comments