other experiments - typically the ones at the Large Hadro Collider; but a hard core of dedicated physicists remains actively involved in the analysis of the 10 inverse femtobarns of proton-antiproton collisions acquired in Run 2, in the conviction that the Tevatron data still provides a basis for scientific results that cannot be obtained elsewhere.
One of the areas where the Tevatron data retain high interest is the measurement of the top-antitop forward-backward production asymmetry. The asymmetry is connected to the fact that the two projectiles at the Tevatron are different, and QCD indeed predicts that a larger number of top quarks are produced in the direction of the proton beam, and antitops in the antiproton direction; at the LHC there is a different effect due to the fact that both projectiles are protons. This makes the Tevatron measurement intrinsically interesting for QCD studies, and necessary as it cannot be replicated at the higher-energy CERN facility; but of course there is more.
In the recent past, CDF has measured a larger asymmetry in top decays than what quantum chromodynamics predicted. Since the production of a high-mass particle decaying to top-antitop quark pairs could affect the asymmetry distribution in a manner not different from what was observed, the result was exciting news and caused an increased effort to look deeper in the phenomenon: it could well be interpreted as the first indication of new physics coming in from the top sector. The DZERO article just published on the Cornell ArXiV is the latest chapter in the resulting saga.
To measure the asymmetry in top-antitop production, one can use different techniques. These depend both on the final state considered to flag top pair production, and on the observable quantity which is studied to extract the asymmetry.
As far as the final state one decides to focus on, this can be either the clean dileptonic final state that results from the decay of both top quarks into lepton-neutrino-b quark jet, or the more frequent single lepton final state that originates from one lepton-neutrino-b jet decay and one three-jet decay of the two top quarks (maybe I should explain that the top quark almost always decays to a W and a bottom quark, and the W in turn decays to lepton-neutrino pairs or jets of hadrons: hence the three-body classification above).
Restricting to electron and muons as charged leptons (the tau is experimentally harder to detect), the dileptonic final state arises 5% of the time, while the single lepton final state arises 30% of the time. Ths results from the fact that the W boson decays one ninth of the time into each kind of lepton-neutrino pair, and two thirds of the time into hadronic jets.
Instead, for an asymmetry analysis the reliance on the most probable (45%) "all-hadronic" final state where both top quarks decay to three hadronic jets is unfeasible, as one then does not have the charged leptons to tell one whether one is looking at a top decay or an antitop decay. It is in fact the electric charge of the lepton what eventually allows one to measure an asymmetry.
Unlike previous analyses, where the top-antitop decay chain has been reconstructed in full using all detected final state objects, DZERO chooses this time to exclusively look at the charged leptons, reasoning that this way they are less dependent on the details of the production model, and that they retain a higher signal efficiency: they can e.g. also make use of the subset of events which features only three jets in addition to the charged lepton and neutrino signatures, which contains a significant amount of signal (one of the final state jets is sometimes lost through the beam pipe or undetected due to failing transverse energy thresholds or merging with another jet) and is usually ignored when attempting a complete reconstruction of the top-antitop decay kinematics.
So DZERO measures the charge and the "rapidity" y of the leptons they can associate to a single lepton top-antitop decay. The rapidity is a function of the polar angle that the lepton makes with the beam axis: zero rapidity corresponds to leptons emitted orthogonally from the beam, and plus and minus infinite rapidity limits coincide with the proton and antiproton directions.
With the number of leptons produced in the two directions, "forward" and "backward" -NF and NB - corresponding to positive and negative rapidity for positively charged leptons (and the opposite if the lepton is negatively charged), one may construct the observable A_FB = (NF-NB)/(NF+NB). Of course, before this number can be meaningfully extracted, one must be able to separate top production events from all backgrounds. It becomes useful to divide the sample of signal candidates into subsets depending on the number of observed jets and the number of b-tags these contain, as the different subsets thus derived contain different fractions of signal.
The separation of background from signal is performed by using those kinematical variables that best distinguish the top-antitop signal from W+jets production, while being well modeled and understood. Of course these variable must have little correlation among them and with the lepton rapidity and charge which enter the definition of the A_FB number. Below are shown four variables used to discriminate top from W+jets in the subset of events with four hadronic jets. The red histogram corresponds to the top signal content, and the green one represent the dominant background from W+jets events; black points are the data from 10/fb of collisions; the lower panels in each of the graphs shows the agreement between data and simulations as a ratio. Similar graphs are contained in the paper for the other categories.
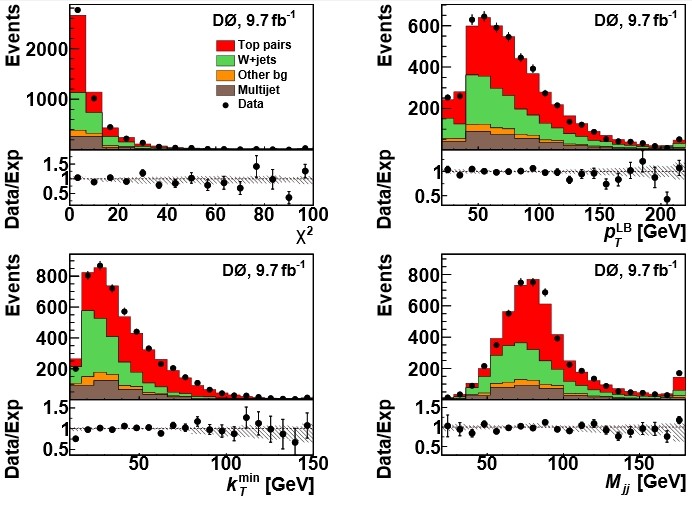
One aspect of the analysis requiring special care is the understanding of the lepton asymmetry provided by the W+jets background: a mismodeling of this contribution to the observed distributions can have a large impact in the final result. DZERO studies the asymmetry in a control sample of data with three jets and no b-tags, which is depleted of top signal, to verify the model of the background asymmetry. A discrepancy between the observed and predicted asymmetry in that sample is corrected with a reweighting procedure.
Finally the measured asymmetry is unfolded in each channel. Unfolding is used to correct the measured distribution such that one obtains the asymmetry of the leptons before detector effects smear it. The corrected distribution can then profitfully compared to simulation predictions at "generator level". I will not enter in a detailed description of the technique used, but here I have a small critique to move to my DZERO colleagues.
In general unfolding is a technique that allows one to obtain from a measured distribution of a random variable the distribution of that variable before the effect of some smearing. That sounds like a free lunch, and indeed it is. The technique works because one takes some assumptions on the smoothness and the general behaviour of the distribution before the smearing. It is a good idea to use unfolding if one wishes to produce a distribution decoupled from the effects of one's detector: a theorist who a few years later comes up with a prediction for the distribution can compare it to the published data without the need to know the details of the detection apparatus.
In the case of the DZERO analysis, however, one sees no reason for the application of unfolding. Much more principled would be to smear the detector distributions before comparing them to the data. The DZERO result is a number (okay a few numbers, as many as the few bins of lepton momentum considered and the different subsets where the signal is isolated). The derivation of that number through a unfolding technique is affected by a systematic uncertainty due to the assumptions taken to unfold. I think this is a perfectible procedure, or at least a unprincipled one.

The four-jets, 1-b-tag subset appears to show a larger asymmetry than the others but we can ascribe that to a statistical fluctuation: it is a 2-sigma discrepancy overall, and we should be happy to see them published nonchalantly in one every twenty papers or so; much more worrysome would be to not see such effects altogether - it would mean that the scientific collaborations block those "ill-understood" results, with resulting biases in the published measurements.
In conclusions, due congratulations to my DZERO colleagues for pulling off this nice precise measurement despite the shortage of manpower. And the wish that in the future they use unfolding techniques only when strictly needed.
Comments