What happens to our brains as we experience the outside world? Scientists have learned that the brain undergoes structural changes as it absorbs sensory data, learns and adapts, but the actual mechanism of this process is just now coming into view.
The following is an edited transcript of an interview provided by the Kavli Foundation, conducted June 10, 2010.
Could you start by giving us a brief preview of what you’ll be covering in your FENS lecture?
What we’re working on in my lab is neuronal plasticity -- how the brain adapts to changes in the environment. Such adaptations can be relatively straightforward. For example, if an animal loses a limb or an eye, the brain adapts to that and partially compensates for the missing information. Other adaptations are more subtle – when, for instance, an animal hides food for the winter and remembers the place later on. Although these things may seem quite different, they have one thing in common, namely that in both cases the brain enables an animal to adapt its behavior because of challenges in its environment.
Over the last couple of years our field has made such fundamental progress that we are now able to really look into the brain and see how it works, in the living animal. We are now able to see the changes related to such adaptations and to see how nerve cells form new connections or how connections between nerve cells are broken. This is basically what I will cover in my lecture. I will talk about our studies investigating what happens when nerve cells make new connections in vitro, i.e. in cell culture. I will on the other hand also talk about our in vivo studies, in which we study the intact organism. We are now able to look into the brain of an animal and see how nerve cell connections are made or broken, and how that relates to learning or other adaptive changes.
How close are you to understanding the mechanism of synaptic plasticity? Is there a particular knowledge breakthrough, a “Holy Grail,” that you're seeking?
Like always in science, that really depends on the level of detail with which one is satisfied. You can always, once you’ve understood something, go further and say, well, we’ve still not completely understood it, so let’s go to the next step. It’s difficult to say how far we’ve gotten. I’d say we’ve understood a couple of fundamental steps. We know, for instance, quite precisely which receptors in the brain are responsible for changes in the functional connections between nerve cells, the synapses; we also know the rules, when synapses get stronger and when they get weaker. All those things are quite well understood and have been elucidated over the last 10 to 15 years or so. But that’s not to say there isn’t plenty of stuff left to be discovered. Science is a never-ending story, which is partly also the fun of it.
In reference to the “Holy Grail,” at least in my personal lifetime or my scientific lifetime, what I would really like to be able to show is that it is really the changes in connections between nerve cells that cause information to be stored in the brain. It would be great to show for instance, that an animal learns something and you see that a thousand synapses change. Then, when you disable those thousand synapses, you see that the animal has forgotten exactly what it had learned before -- but nothing else.
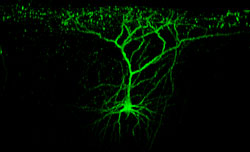
A two-photon microscope image of a layer 2/3 nerve cell in the visual cortex of a mouse. Tobias Bonhoeffer and colleagues use two-photon microscopy, which allows imaging to a depth of approximately half a millimeter, as one method of studying structural changes in the brains of living animals. The technique uses infrared lasers to excite fluorescence in intact tissue. Copyright: MPI of Neurobiology / Huebener.
It appears from much of your published research that advances in the technology of imaging have been central to your work. Could you say a little bit about where imaging is going and what might be the next step?
The advent of new microscopic techniques over the past 10 to 15 years or so has really made a huge impact. On one side, microscopes are important. But equally important are the advances in producing the molecules that actually make the nerve cells visible, that is fluorescent. In particular, novel techniques make such molecules genetically encodable -- which means that they need not to be introduced into the cells, but are then produced by the cells themselves. This is now possible and a fantastic advance that technology has made over the last few years. It enables us, in a living animal, to make all nerve cells, or a subset of them -- for example only the inhibitory or only the excitatory cells – visible through fluorescence. We can then observe these cells with the new microscopes for days, or even for weeks or months.
In addition -- and this is also relatively new -- one can use genetically encoded probes also to study the activity of nerve cells. Not only can you see how their structure changes, but you can at the same time see how they react to specific stimuli and perhaps how their reaction changes over weeks when the animal adapts to its environment. All of this can be done in the living animal, and that’s a huge step forward compared to what was possible only a couple of years ago.
So it seems that, with this new technology, the entire brain is largely accessible to imaging now, whereas with earlier technology you could only get to a shallow layer on the surface of the living brain. So now can you get deep into it?
You can, but it’s still an advantage if you deal with the surface. There are tools with which you can really look into it, yet they all have their disadvantages. The good thing is, though, that the cerebral cortex, which is the part of the brain that harbors the higher cognitive functions, is on the outside of the brain. So it’s relatively easy to access this structure optically, especially in a brain with a structure like a mouse's, which doesn’t have the deep folds that for instance a human brain has. You can see down into the tissue for approximately a millimeter which is where many of the most interesting things occur.
Neuroscience is clearly a field with plenty of input from other disciplines. Which of those disciplines are feeding into it most -- and perhaps changing it?
That’s a very hard question to answer. It depends very much on the point of view. I’ll give you an example. The stuff I do certainly needs physics and cell biology in order to study cells with the advanced microscopy and the genetically encoded fluorescent molecules that I mentioned. So I use biosciences plus physics and engineering. But that’s only for me. Other people are actually going much more into the cognitive direction – psychology, even humanities. For example, at the Max Planck Society, we will soon be founding a new institute on “empirical aesthetics.” The idea is to find out what makes the human find something aesthetic – be it music, literature, art, whatever. In this case, where you try to understand what makes a human brain find something aesthetic, you will cooperate with people working from brain science all the way to humanities. So there’s a huge span of things over which neuroscience can interact with other disciplines. I gave you the physics and engineering example on one side, but you can also talk about music and literature on the other side.
And it sounds like nanoscience has a huge effect on the tools one is using.
Absolutely. I’m actually just travelling to a McKnight Conference [in Aspen, Colo.] where I’m on a panel which is judging and awarding the technological innovations that are going to change neuroscience in the future. On the plane, I read a couple of applications centered on nanoscience, where tiny tubes are etched into silicon wafers to get substances into the brain or outside of the brain, or even to have better access to single cells and to get substances in and out of cells. So there’s no question that nanoscience is very important.
Is your work pointing toward medical advances -- toward cures for neurological degenerative diseases like Alzheimer’s, Parkinson’s and so forth?
That's not easy to answer at this point. Basic science is always going to be good for medical applications, because for curing any brain disease it will be very helpful to understand how the normal brain functions. Thus, by studying and understanding how memory is formed or lost, we provide a basis for later studies towards treatments of such diseases. However, I wouldn’t say, particularly in respect to a disease like Alzheimer’s, that due to our work we are close to curing memory loss. Something as big as that will take many more years of study. We know, for instance, that particular receptors are central to learning and the storage of information. But if you start to manipulate them, there are many side effects such as epilepsy. So we need to be very cautious and patient in order to make progress with curing diseases such as the ones you mentioned.
The power of sensory experience to influence the structure of the brain raises a question: Does technology -- for instance, in the way we use it, through media, computers and so forth – have such an affect on how the brain develops that we might have substantially different brains than, say, people did 150 years ago?
Absolutely. I’m 100% convinced of that. But at the same time I don’t necessarily find it scary, because – to give you an example – the brain of someone who has learned to play the piano will also be different from somebody who had not learned to play the piano, and nobody would worry about that. Whenever you learn something - a language, new motor skills, to play baseball, whatever – it will change your brain, and that’s not necessarily something bad. Everything that you experience will change your brain. One example -- and people who are bilingual or trilingual know this -- you think differently in different languages because there are different expressions.
For instance, in German there is a word, “Himmel,” that means both “sky” and “heaven.”. I remember, when I was a kid, for me there was no difference between sky and heaven, it was the same thing. Only when I learned English and was taught these two words, I thought more about it and realized that they were in fact two different things. There are multiple examples of that sort, of course. The essence is that if you use a particular language, it will change the way you think – it will change your brain. The same is true for everything else we do.
How does your research shed light on how much of the brain’s structure is genetically determined, and how much develops through experience? Do you have insight as to what degree the “blank slate” idea is true or untrue?
We worked on that question some years ago and I am still quite interested in it. The “blank slate” idea certainly is not true; I think most neuroscientists would agree to that. But the question of what percentage of the brain's structure and function is based on genetic predisposition or on experience doesn’t have a clear-cut answer. Different people will have different opinions about that.
I personally believe, from the experiments that we have done, but also just from looking at my kids or myself, that a huge amount is actually genetically determined. Many people observe that, when they grow older, whether they like it or not, they become more like their parents. For me, that’s one of the many indications that lots of things are predetermined. Of course, that's not to say that one should give up educating kids.
Looking ahead 10 or 20 years, what would you guess the state of the science would be then, the state of our knowledge and the state of our ability to deal with the brain or even manipulate it?
First of all, in terms of mechanistic details – the stuff I’m working on – there will be a huge amount of progress. The speed with which technologies and ideas develop is really breathtaking. I think that we will be able to do what we do now in much more detail and more precisely and for longer times. We’ll be able to look into the intact brain in a behaving animal and observe the changes that were conventionally only investigated in the dish. On the cognitive side, people are starting to work on the question of emotions, for instance to try to delineate and understand the parts of the brain that are related to empathy. We will see much more of that and I would not be surprised if we would in the next 10-20 years get major insights in areas like this.
Just consider, one could then predict, what happens if such brain areas where underdeveloped. Would this lead to the development of a violent personality? There are many very fascinating questions, which are however also associated with severe ethical concerns. Would one want to scan the brain of somebody, when you suspect that there’s a particular area that’s underdeveloped, or that a particular neurotransmitter isn’t expressed properly? And what would be the consequences of such a scan? Could there be the possibility to use our better understanding of these processes, to cure such deficits based on neuroscientific progress?
Fascinating and problematic at the same time! But this is not the only area in science where discoveries can be used for good or worse. The trick will be to use the scientific possibilities in an ethical and generally beneficial way.
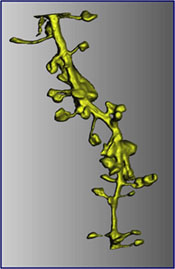
Reconstruction of a dendritic section as seen through simulated emission depletion (STED) microscopy, one of the advanced imaging techniques used by Tobias Bonhoeffer and his colleagues to show structural change in the brain. This high-resolution 3D image was assembled from multiple images at depths separated by 0.25 μm. Copyright: MPI of Neurobiology / Bonhoeffer.
One more question about your science and its future: What possibilities excite you most as you conduct your research?
That brings me back to one of the first questions, where we talked about the Holy Grail. It would be great to really understand how the information storage works in the brain. It would be fascinating if you could look into an intact brain with those new technologies and see how new connections are being made while an animal learns something. Then, if you could actually show that the loss of those connections results in the animal forgetting what it learned, you would have the first really good evidence that you have understood an important component of memory and information storage.
To me, that would be fantastic, because I think memory is one of the most important properties of the brain. Without memory we could move and react to the environment, but we could never adapt to changes and would thus be much worse equipped to survive in an ever changing environment. Memory for me is a fundamentally important phenomenon in biology. If we could contribute to understanding and resolving some of its mystery I would be very happy.
Comments