It should be clear by now that both the fusion and fission processes can generate energy - the trick is to make these processes sustainable. While I am keeping my fingers crossed that we can produce a viable means for generating electricity with fusion, I personally do not think this will happen within the next 20 to 30 years. I think one of the areas of active research should be on fusion power. The main issues with fusion is the large temperatures required to actually overcome the repulsive forces between the nuclei of atoms and the utilisation of the released energy in generating electricity. Although I have a vested interest in fission power, I think that if fusion becomes mature and viable, it is possible that it could overshadow all existing means of power generation - whether or not this will happen, we will have to wait and see. With this, I'd like to end my discussion on fusion power. There are several good introductory sources of current fusion projects and research that can be accessed via the Internet. An especially interesting one is the ITER project, and I would recommend anyone interested in the topic to keep your eyes open for the outcomes of these experiments.
Fissionable and Fertile materials
Fission power on the other hand is a mature and very well understood process. As mentioned previously, heavier atoms have lower binding energy than the peak of around 8.7MeV for Iron and Nickel. Uranium, the heaviest naturally occurring element has a binding energy of around 7.5MeV. There are 2 naturally occurring isotopes of Uranium, U-238 which accounts for about 99.3% of terrestrial uranium, and U-235 which accounts for the remaining 0.7% (the numbers 238&235 represent the sum of the protons and neutrons in the nucleus - since they are both the same element, they have the same number of protons, but differing number of neutrons). Not all atoms are equally stable as mentioned earlier. differing number of neutrons affect its stability. In the case or Uranium, U-235 has a shorter half-life than U-238, and therefore decays faster than U-238. The result is the high natural occurrence of U-238 compared to U-235. So why is this important? For starters, an interesting property that has been observed for heavy nuclei is that odd numbered isotopes are easier to fission than even numbered isotopes.
So, for the purposes of getting a sustainable fission reaction, we need to focus on U-235. The U-235 is known as the fissionable isotope while the U-238 is known as a fertile isotope.
Now I did mention earlier that one method that an atom decays is through spontaneous fission. While this is an important property of some atoms, engineers do not rely on this to get fission going, instead we rely on neutrons. As mentioned before the number of neutrons determine to a large extent the stability of an atom. Therefore, bombarding a fissionable material with neutrons will get the fission process going. When the atom fissions, it splits into 2 usually unequal pairs of highly excited by-products. Together with this, a number of additional neutrons are released. Therefore, if more neutrons can be produced than consumed for a fission reaction, then you have a chain reaction - this is the basics of what goes on inside a reactor or a nuclear bomb. The main difference between the 2 is that in nuclear bombs, the chain reaction is unchecked and allowed to propagate as much as necessary to create the ultimate explosion, while in reactors the reaction is controlled by various natural and man-made safety measures.
In the case of nuclear bombs, very highly enriched uranium or plutonium (only fissionable isotopes) are used - this is so that most of neutrons released during a fission will be captured by another fissionable atom and continue with the chain reaction. When you incorporate fertile isotopes such as U-238 into the reactor, these non-fissionable atoms capture some of the neutrons and will not produce as much "bang for your buck". Of course, when U-238 captures a neutron, it temporarily becomes U-239 and undergoes a chain of beta decays into Pu-239 - the other main nuclear weapon fuel. It should be clear now why U-238 is know as fertile. It is able to produce more fissionable isotopes. It should be noted that U-238 can itself fission if the neutron that it captures is of high enough energy, but this can be mostly ignored for a general discussion about fission reactor physics.
Cross-Sections
Now another aspect that comes into play is the fact that the energy of the neutron affects how if reacts with an atom. In a very high level discussion, you can consider that when a neutron encounters a nucleus, 2 types of reactions can occur. These are scattering and absorption. Under absorption, you have the option of either fission or radiative capture. With radiative capture, the atom simply absorbs the neutron and emits a gamma particle. Now, the chances of each of these reactions happening is depended on the energy of the incident neutron as well as the atom that it is interacting with. Graphs of these for almost all relevant atoms have been plotted and are known as its cross-sections. An example fission cross-section for U-235 can be found at http://t2.lanl.gov/tour/u235nf.gif.
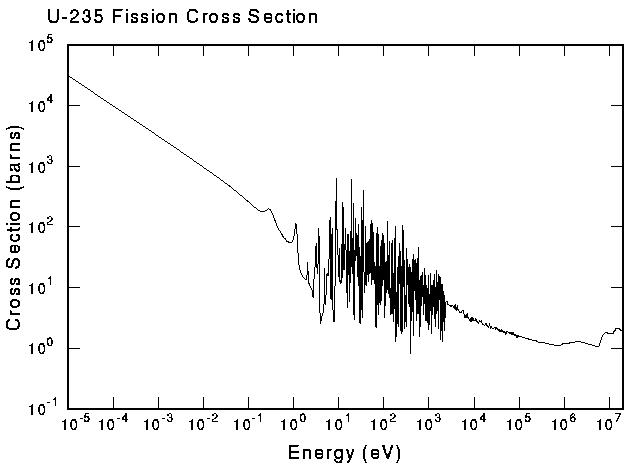
Let me take a moment to explain what the cross-section represents. Since neutrons are charge-less particles, they are not attracted or repelled by the electromagnetic forces of an atom. It is easy to therefore think that neutrons will travel in relatively straight lines until it decays or physically encounters the nucleus of another atom. While this specific model is accurate for 1 certain type of reaction (elastic potential scattering), it is in general not accurate. While the nucleus of an atom is very small, the neutron effectively sees a much larger area to collide into for each type of reaction. This effective "size" of the nucleus in attracting a neutron to produce a reaction is what is measured by the cross-section. Take for instance the fission cross section of U-235; at low energies the size of the cross section is magnitudes of order larger than the actual size of the nucleus which is one of the reasons why fission power is possible. It is a difficult concept to grasp, but probably one of the most essential aspects of fission physics. Perhaps another way of looking at it is as a measure of how badly the atom wants to attract a neutron to produce a certain reaction.
Now taking a more detailed look at the fission cross section of U-235 we can see that the magnitudes are quite large and smooth at lower energies, a bit haphazard at intermediate energies and rather small but also smooth at very high energies. Scientists and engineers like smooth graphs, mainly because it is easier to predict what will happen. This is one of the reasons why intermediate energies are avoided in reactor physics. Also, since the fission cross-sections are so high at lower energies, we try and ensure that the reactor fission reactions occur mainly in the low energy region. Conversely, for nuclear bombs, most of the reactions occur in high-energy region of the graphs. I would like to introduce a few common nomenclature before continuing.
Firstly, the low energy region is generally known as the thermal region; the intermediate energy region with all the haphazard peaks are known as the resonance region and the high energy region is known as the fast energy region. These naming conventions are used in describing the different types of reactors that are available. Another point to note is that the U-235 cross-section graph can be used as an archetypal example of what such a cross-section for most fissionable materials will look like - the main difference would be in the magnitude of the graph and the locations of the resonances.
Moderation
Moving along, it must be noted that neutrons that are produced in a fission reaction are usually very energetic and therefore appear in the fast energy spectrum. While fission can occur at these energies, it is much easier for a neutron to be captured and for a fission reaction to occur at thermal energies. Therefore, a means to slow the neutrons down to such energies are needed. This process is known as moderation, and plays a very important role in thermal reactors. Moderation allows for fission to occur in relatively low concentrations of U-235. So what is moderation? Well, in its simplest conceptual form, the neutron energy relates to its speed - which relates to a high energy neutron moving very fast. Therefore, by using Newtonian mechanics we can slow down the neutron (and thereby reduce its energy) by having it bump into other objects and transferring some of its kinetic energy over to the material it is bumping into. But to be efficient in this process, we need to have the neutron potentially lose as much energy in each collision as possible.
Now, if the nuclei that the neutron is bumping into is large, then it would not lose a lot of energy in each collision - so it makes sense to have the moderator's atomic mass to be as low as possible to increase its' effectiveness as a moderator. And this is usually the role that water plays inside a reactor. Since the Hydrogen atoms are the smallest you can get, it can most easily transfer the kinetic energy away from the neutron. However, water is generally not the best moderator that can be used because the oxygen in the molecule has a non-zero absorption cross section and therefore it captures some of the neutrons it is trying to slow down. And the number of neutrons is the main aspect of the reactor that an engineer tries to control. The following is a list of moderators based on how well they can moderate and how little they absorb neutrons: Heavy water (where the hydrogen is H-2 and not H-1), graphite and only then Water.
So how do reactors operate
So now that you know all of that about fission, it is finally time to paint the whole picture. You usually have a mixture of Fuel (typically slightly enriched uranium) and moderator in the reactor core. The reaction is started off either by an external neutron source or by slowly removing the control rods. The produced neutrons are then captured by atoms of U-235, whereby they undergo fission. This fission produces more neutrons than was consumed. These are fast, energetic neutrons and typically do not interact with the fuel but rather reach the moderator where they are bounced around and eventually thermalised. These thermalised neutrons find other atoms of U-235 and the process continues. The moderator in the case of Light Water Reactors (LWRs) also act as the coolant for the core. Most of the energy released by the reaction is in the form of kinetic energy in the fission products.
Due to the nature of the fuel cell, these fission products do not travel too far, and heats up the fuel cell. The water carries the heat away from the fuel cell to a steam generator which will then produce steam to produce electricity. Each fission reaction typically produces around 200MeV of usable energy that the coolant carries to the steam generator. Once the required power level of the reactor is reached, then control rods and chemical additives help to regulate the neutrons produced and maintain the stability of the reactor.
I hope the basic physics for nuclear fission has been explained in this post. If more information is needed, please ask.
Comments