Continued from Part 2, A Scientific Approach to Science Education - Research On Learning
On average, students have more novicelike beliefs after they have completed an introductory physics course than they had when they started; this was found for nearly every introductory course measured. More recently, my group started looking at beliefs about chemistry. If anything, the effect of taking an introductory college chemistry course is even worse than for taking physics.
So we are faced with another puzzle about traditional science instruction. This instruction is explicitly built around teaching concepts and is being provided by instructors who, at least at the college level, are unquestionably experts in the subject. And yet their students are not learning concepts, and they are acquiring novice beliefs about the subject. How can this be?
Cognitive scientists have spent a lot of time studying what constitutes expert competence in any discipline, and they have found a few basic components.
The first is that experts have lots of factual knowledge about their subject, which is hardly a surprise. But in addition, experts have a mental organizational structure that facilitates the retrieval and effective application of their knowledge. Third, experts have an ability to monitor their own thinking (“metacognition”), at least in their discipline of expertise. They are able to ask themselves, “Do I understand this? How can I check my understanding?”
A traditional science instructor concentrates on teaching factual knowledge, with the implicit assumption that expert-like ways of thinking about the subject come along for free or are already present. But that is not what cognitive science tells us. It tells us instead that students need to develop these different ways of thinking by means of extended, focused mental effort. Also, new ways of thinking are always built on the prior thinking of the individual, so if the educational process is to be successful, it is essential to take that prior thinking into account.
This is basic biology. Everything that constitutes “understanding” science and “thinking scientifically” resides in the long-term memory, which is developed via the construction and assembly of component proteins. So a person who does not go through this extended mental construction process simply cannot achieve mastery of a subject.
When you understand what makes up expert competence and how it is developed, you can see how cognitive science accounts for the classroom results that I presented earlier. Students are not learning the scientific concepts that enable experts to organize and apply the information of the discipline, nor are they being helped to develop either the mental organizational structure that facilitates the retrieval and application of that knowledge or a capacity for metacognition. So it makes perfect sense that they are not learning to think like experts, even though they are passing science courses by memorizing facts and problem-solving recipes.
Improved Teaching and Learning
If we now return to the puzzle of my graduate students—why their first 17 years of education seemed so ineffective, while a few years of doing research turned graduate students into expert physicists—we see that the first part of the mystery is solved: Those traditional science courses did little to develop expert-like thinking about physics.
But why is working in a research lab so different?
A lot of educational and cognitive research can be reduced to this basic principle: People learn by creating their own understanding. But that does not mean they must or even can do it without assistance. Effective teaching facilitates that creation by getting students engaged in thinking deeply about the subject at an appropriate level and then monitoring that thinking and guiding it to be more expert-like.
When you put it in those terms, you realize that this is exactly what all my raduate students are doing 18 or 20 hours a day, seven days a week. (Or at least that is what they claim—the reality is a bit less.) They are focused intently on solving real physics problems, and I regularly probe how they’re thinking and give them guidance to make it more expert-like. After a few years in that environment they turn into experts, not because there is something magic in the air in the research lab but because they are engaged in exactly the cognitive processes that are required for developing expert competence.
Once I realized this, I started to think how these ideas could be used to improve the teaching of undergraduate science. Of course it would be very effective to put every student into a research lab to work one-on-one with a faculty member rather than taking classes. While that would probably work very well and is not so different from my own education, obviously it is not practical as a widespread solution.
So if the economic realities dictate that we have to use courses and classrooms, how can we use these ideas to improve classroom teaching?
The key is to get these desirable cognitive activities, as revealed by research, into normal course activities.
I am not alone in coming to this conclusion. There is a significant community of science-education researchers, particularly in physics, who are taking this approach to the development and testing of new pedagogical approaches. This is paying off in clear demonstrations of improved learning. Indeed, some innovative pedagogical strategies are sufficiently mature that they are being routinely replicated by other instructors with similar results.
So what are a few examples of these strategies, and how do they reflect our increasing understanding of cognition?
Reducing Cognitive Load
The first way in which one can use research on learning to create better classroom practices addresses the limited capacity of the short-term working memory. Anything one can do to reduce cognitive load improves learning. The effective teacher recognizes that giving the students material to master is the mental equivalent of giving them packages to carry.
With only one package, they can make a lot of progress in a hurry. If they are loaded down with many, they stagger around, have a lot more trouble, and can’t get as far. And when they experience the mental equivalent of many packages dumped on them at once, they are squashed flat and can’t learn anything.
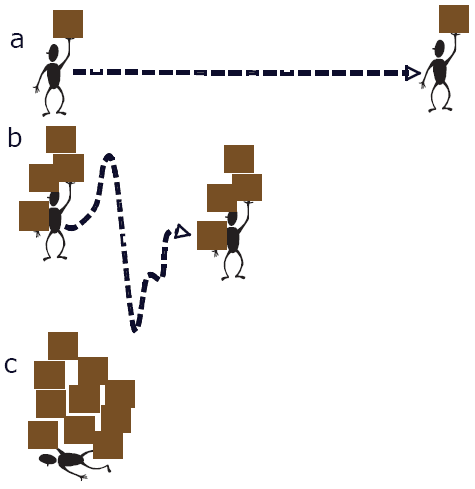
Students with low, medium and high cognitive loads.
We'll address beliefs,guided thinking and technology in Part 4.
REFERENCES:
W. Adams et al. (2005), Proceedings of the 2004 Physics Education Research Conference, J. Marx, P, Heron, S. Franklin, eds., American Institute of Physics, Melville, NY, p. 45.
R. Hake (1998), The American Journal of Physics. 66, 64.
D. Hammer (1997), Cognition and Instruction. 15, 485.
D. Hestenes, M. Wells, G. Swackhammer (1992), The Physics Teacher. 30, 141.
Z. Hrepic, D. Zollman, N. Rebello. “Comparing students’and experts’ understanding of the content of a lecture,” to be published in Journal of Science Education and Technology. A pre-print is available at http://web.phys.ksu.edu/papers/2006/Hrepic_comparing.pdf
E. Mazur (1997), Peer Instructions: A User’s Manual, Prentice Hall, Upper Saddle River, NJ.
G. Novak, E. Patterson, A.Gavrin, and W. Christian (1999), Just-in-Time Teaching: Blending Active Learning with Web Technology, Prentice Hall, Upper Saddle River, NJ.
K. Perkins et al. (2005), Proceedings of the 2004 Physics Education Research Conference, J. Marx, P. Heron, S. Franklin, eds., American Institute of Physics, Melville, NY, p. 61.
E. Redish (2003), Teaching Physics with the Physics Suite, Wiley, Hoboken, NJ.
*****
Originally presented in Change magazine, September/October 2007.
Comments