How a fish ‘broke’ a law of physics
... says a press release from Bristol University.
A great credit to the French is how, amid the turmoil of their revolution, so many of their scientists and mathematicians continued to come out with great discoveries (provided that they managed to keep their heads, unlike Antoine Lavoisier.) Among their number was Étienne-Louis Malus (1775 – 1812) who served with Napoleon’s army in Egypt, but on his return to France developed his work in optics. They didn’t have Polaroid in those days, but they did have Iceland spar crystals, whose properties had been studied over a century earlier by Christiaan Huyghens, a contemporary of Newton. Malus was looking through a crystal at light reflected from the windows of the Luxemburg Palace in Paris, and noted the changes as he rotated the crystal. So doing, he discovered polarization by reflection, and invented the term polarization itself.At a particular angle, Brewster’s angle, the reflected light is completely polarized. Looking at the diagram, it is appears that light which is polarized to vibrate along the direction of view (dots) is at least partially reflected, but light polarized perpendicular to this (arrowheads) is not reflected at all. This would reduce reflectivity.
Now if one is a small silvery fish, it might be advantageous to overcome this effect, so that light reflects in all directions. Then one could blend better against the light coming from the sky, especially as seen be something big, swift and hungry.
In the last century, we have learned to play lots of tricks with light by use of special optical coatings. Ever since the invention of the telescope, a way has been needed to reduce reflection at the surfaces of lenses. With Galileo’s telescope, there were only four surfaces between sky and eye. But with modern compound lenses in telescopes and cameras, with many elements to reduce aberration, there are many surfaces through which the light has to pass, not to mention those of the prisms in binoculars and single lens reflex cameras. Reflection in these systems is greatly reduced by coating the surfaces with thin films of materials of different refractive index, so that interference can ‘block’ the reflected beams and so ‘force’ the light to go through in the desired direction.
Even more extreme behaviour had be achieved by use of multilayers, which can give effectively complete transmission, polarization or absorption, whichever is desired. Examples can be seen in the (admittedly commercial) online Photonics Handbook .
But can one design a multilayer to be effectively ‘not there at all’? It seems that some fish have achieved this. People have only been systematically deep sea fishing for, I would guess, a few thousand years, but marine predators have been in on the act for hundreds of millions. It seems that some of our favourite food species have come up with a method of camouflaging themselves in the sea.
Three species have been studied by the Bristol group, namely the Atlantic herring (Clupea harengus), the European pilchard (Sardina pilchardus), and the European sprat, (Sprattus sprattus — now that and the previous name sound like fine old Latin!).
The skins and scales of these fish contain highly reflective multilayers made of — in the skin — cytoplasm and mixed crystals of guanine (the G in DNA) and hypoxanthine. These crystals are highly optically anisotropic, with the refractive index difference between the c-axis (1.46) and the a and b-axes (1.85, 1.81) nearly three times that of Iceland spar, the classic ‘polarizing’ crystal.
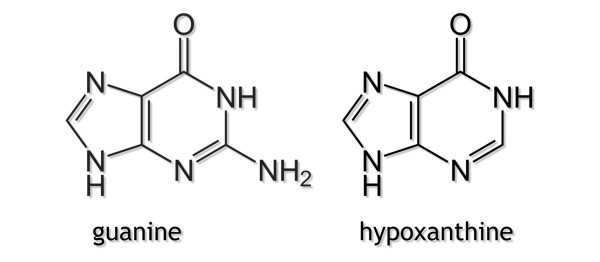
These things being known (as Julius Caesar would say), one would expect the reflective fish skin to be highly polarizing indeed. But this is not the case. On picking apart the skin and studying individual crystals under a digital holographic microscope, it becomes apparent that there are two distinct types for crystal, chemically very similar but optically quite different.
Using computer modelling, they generated the following structure to match the optical properties of the fishy skin:
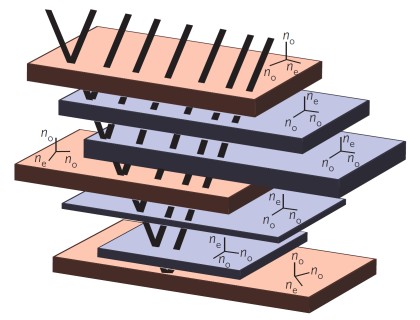
The refractive index axes are labelled no (ordinary) for the two similar high indices and ne (extraordinary) for the much lower index. In type 1 crystals (blue-purple) the extraordinary direction is perpendicular to main plane of the crystal, whereas in the type 2 crystals the extraordinary axis and one ordinary axis are randomly located in the main plane. The crystals also vary in thickness, which would avoid the multilayer behaving selectively for different angles of reflection or wavelengths of light.
So even if a predatory fish, cetacean, or cephalopod were taking things one step further, and relying on built in polaroids in their eyes to help them hunt, then in this matter at least the herrings and their relatives would be one step ahead in that particular arms race. Dr Nicholas Roberts, group leader, says:
“We believe these species of fish have evolved this particular multilayer structure to help conceal them from predators, such as dolphin and tuna. These fish have found a way to maximize their reflectivity over all angles they are viewed from. This helps the fish best match the light environment of the open ocean, making them less likely to be seen.”
However, these species are now heavily predated by humans employing nets cast from trawlers. So what would be the next step? One science fiction writer (Richard Cowper) had a main character wondering if the rectangular shape of fish fingers may have been naturally selected as the optimum for escaping through the mesh of a net.
Paper: ‘Non-polarizing broadband multilayer reflectors in fish’ by T.M. Jordan, J.C. Partridge and N.W. Roberts in Nature Photonics DOI: 10.1038/NPHOTON.2012.260
Comments