We have long known that many of the Earth's rocks are formed by the solidification of molten rock. Since James Hutton wrote his 1788 book 'The Theory of Earth', granite has been acknowledged to be formed by the solidification of molten rock. Since then, large granitic bodies, along with their andesitic and gabbroic equivalents, have been recognised as 'fossil magma chambers'. Formed deep underground and then uplifted and eroded, they have provided valuable information on their current-day relatives beneath active volcanoes. Along with the study of volcanic products — and more recently geophysical methods — they have lead to an understanding of the processes that go on beneath volcanoes, although that understanding is far from complete.

"Upon the whole, therefore, whether we shall consider granite as a stratum or as an irregular mass, whether as a collection of several materials, or as the separation of substances which had been mixed, there is sufficient evidence of this body having been consolidated by means of fusion, and in no other manner." James Hutton. Picture: Detailed East-West Section, Northern Granite, Isle of Arran, Strathclyde USGS Multimedia Gallery.
Discussions of magmatic processes often seem a little academic, especially when viewed in isolation. The relevance to eruptions of many of the questions geologists have asked about the subsurface may not be immediately apparent. If you want to predict eruptions, however, it is essential to know what goes on at depth. This post will contain a lot of jargon, but please bear with me. None of the concepts I will discuss are particularly difficult, and I hope by peeling away at the jargon that obscures them I can set the scene before discussing my own research in a future post.
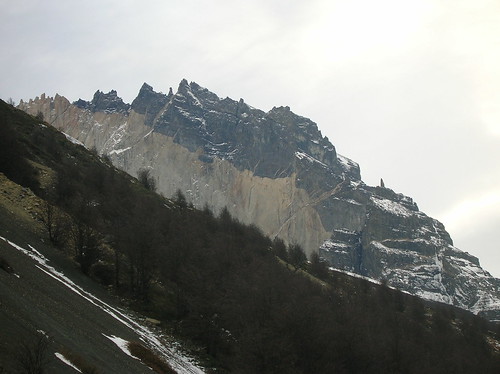
The Torres del Paine fossil magma chamber. The boundary between the granitic magma chamber (the light coloured rock) and the surrounding 'country' rock (dark coloured). Note the fractures, where granitic material has been injected into the country rock.
Solidified magma chambers, or plutons as they are often referred to by geologists, have provided much of what we know about deep volcanic processes. One of the classic sites, one that much of the early work on magma chambers to date has been done on, is Skaergaard in Greenland. This gabbroic intrusion has been rotated slightly, allowing geologist to walk over a slice from what was originally the roof to the floor. What makes Skaergaard particularly useful for studying is its simplicity; the magma was was emplaced and then left to cool. None made it to the surface, and no new magma was injected. Its slow, tranquil construction allowed the processes taking place to be recorded. In the case of Skaergaard, the dominant process was fractional crystallisation.
Magma, like its solid counterpart (rock) is chemically very complex. Its biggest chemical constituent (usually) is silica, or SiO2. Other important elements include iron, magnesium, aluminium, calcium, sodium and potassium. There are also dozens of others, and current technology can measure traces of them of only a few parts per million (ppm). These make up various minerals (such as quartz, olivine and feldspars), which in turn make up the rock.
Certain minerals, such as olivine, have a higher melting point, so as the magma cools these crystallise first. The same thing can happen if you leave a bottle of wine in the freezer for too long. The water will freeze at 0ºC, but the alcohol will stay liquid much longer. This is slightly complicated in magmas, as each mineral is a mixture of chemicals. However just as the wine becomes more alcoholic as more water freezes, the melt in a magma chamber becomes more silicic as more crystals form. The first crystal to solidify are usually low in silica and high in iron and magnesium.
This is just the first part of the story. If the crystals just stayed floating in the magma, its overall composition wouldn't change. If, however, the crystals are separated from the melt, we would see it 'evolve'*. Separating the melt from the crystals is known as fractionation, hence 'fractional' crystallisation. This can be done in many ways. The crystals themselves can be removed, either by sinking to the bottom or floating to the top of the chamber (depending on their density). On Skye, in Scotland, an old, cold magma chamber has been exposed at the surface. Here many sedimentary structures — such as ripples — are seen in the crystals along the bottom of the chamber. These show that not only did the crystals settle out of the magma, but that they formed flows and currents along the bottom.
The other way of fractionating magma is fix the crystals and then remove the melt. Crystals often form on the edges of the chamber, where it is cooler and the crystals have a surface to grow on. Sometimes the growing crystals form a rigid framework. The evolved melt can then be extracted, leaving the crystals behind. In Skaergaard the melt was never removed, but we can still see the effect of fractional crystallisation. Crystallisation started at the outside and worked its way in, so now as we do the same we can recreate its chemical evolution.
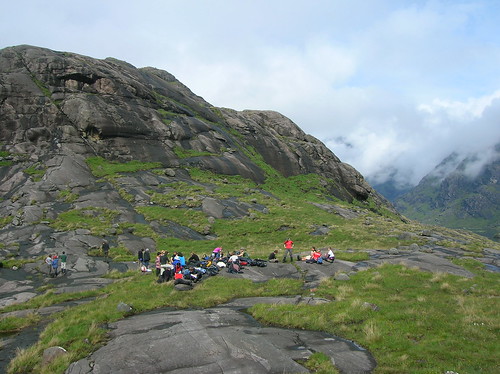
The Coulins, on the Isle of Skye, Scotland. Like Skaergaard they are gabbros formed by the slow solidification of a magma chamber.
When the mantle melts it usually produces a basalt, a type if rock/melt low in silica. Mantle melting is the most common source for the magma at volcanoes (although there are others). Through fractionation, these basalts become first an intermediate (intermediate silica content) andesite, then an evolved, high silica rhyolite. While there are many, many names for rocks of slightly different compositions, generally most differences in behaviour of magma come from how much silica it contains. There are also many names for describing how evolved a magma is, which although they have subtly different meanings. Basalts are variously described as basic, primitive or mafic, while rhyolites can be acidic, evolved or silicic. Another confusion can arise because gabbro is chemically the same as basalt, just with larger crystals. Granite is the large crystal (coarse-grained) version of rhyolite.
Crystal fractionation is not the only process to affect the composition of the melt. The heat will melt the surrounding (or country) rock, which will contaminate the magma. This assimilation has much the same effect as fractional crystallisation. The first minerals in the crust to melt, those with the lowest melting point, will be the same type as the last ones to crystallise from the magma. These added melts (crustal contaminants) will drive the chemistry of the magma towards more silica-rich compositions.
Because of the similarity in the effects of these two processes there was for a long time arguments over which process produced the most evolved magmas, and most destructive eruptions. While we have seen that partial melting and fractional crystallisation can effect the chemical composition of the melts produced, different isotopes of the same element should behave the same during these processes. The ratio of isotopes like 16O/18O (that is oxygen with atomic weights of 16 and 18 respectively), or 86Sr/87Sr (strontium) should stay the same. By measuring the isotopic composition of both the crust near the volcano and primitive, unfractionated magmas, we can calculate how much of the erupted rhyolites were produced by fractionation/crustal melting. For almost all volcanoes fractionation is found to be much more important. There are, however, many large granite bodies around the world formed from crustal melts, but these melts rarely seem to make it to the surface.
So why does all this matter? The most obvious reason is that the composition, and mainly the silica content, has a large impact on the eruptive style. Basaltic eruptions tend to be rather gentle, think Hawaii or Stromboli. Silicic magmas are more sticky or viscous, and trap volcanic gases. This leads to explosive eruptions, like Mt St Helens and Pinatubo. Understanding how these explosive magmas are created, especially in the huge volumes found in the largest eruptions (Yellowstone, Fish Canyon, Taupo) will help us predict where and when the next one will blow. The processes I've mentioned here have been pretty well studied, and now make up the starting point for any studies on a volcano. In a future post I will write about some of the more recent developments and open questions about magma chambers, mainly how fast are they constructed and how long do they last. Traditionally it has been assumed that the largest chambest must have taken hundreds of thousands of years to build, but recent results have begun to change that view. My supervisor has a paper in press about this, so as soon as it is published I will write something about it here.
*Note that in this context the word evolution has very little to do with Darwin's theory of natural selection.
Comments