One word of warning is needed: beware, the list is incomplete, its order is little less than random, and the items do not necessarily correspond to signals of new physics that are giving hints of being present in the data at the time of writing. There are, it is true, two-sigma discrepancies here and there, but who cares about two standard deviations nowadays ?
And another note: the plots I included below are thumbnails, and have not been commented in the text for brevity. You can click on them to get full-size pictures; an explanation is usually provided in the links I have put in the text.
1) Measurement of anomalous gauge boson couplings
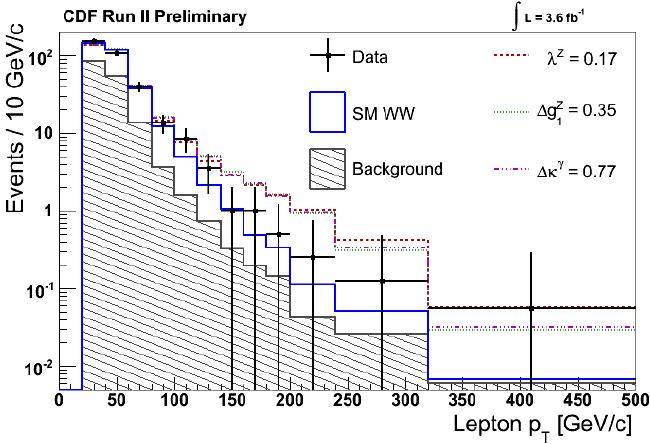
2) Observation of a new Z' boson
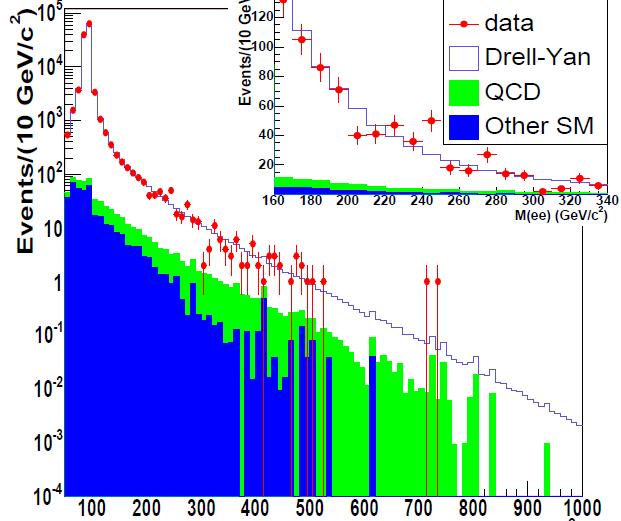
3) Confirmation of anomalous muons, and electrons

4) Observation of a new t' quark
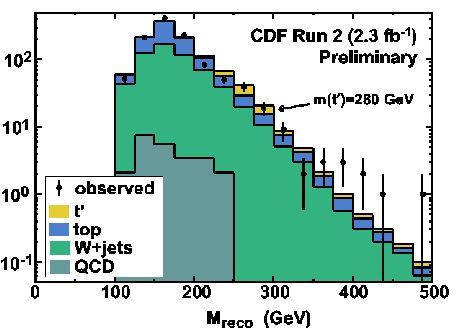
5) Measurement of a strong phase in B_s mixing

6) Observation of large branching fraction of B_s mesons to muon pairs

7) Evidence of large extra dimensions
CDF and DZERO are searching for events where a particle disappears in another dimension. This would provide a striking signature, and would be easily recognizable as new physics. I discussed recent searches of this kind here.
8) Observation of exotic hadrons
Of course, the discovery of molecular states, pentaquark hadrons, etcetera would be exciting; it is not clear, however, that these might be ascribed to Physics beyond the standard model, since quantum chromodynamics contains no definite rule against such states.
10) Observation of top-antitop resonances
If you have a large sample of top-antitop events, you may be tempted to reconstruct the total mass of a hypothetical particle X which decayed to produce the two top quarks. The Tevatron experiments have been doing this for a while now, but apart from some early excitement, the mass distribution of X candidates appears to closely follow expectations - for the time being.
11) Observation of a Supersymmetric Higgs boson
If Supersymmetry (SUSY) is the theory, then one of its five Higgs bosons might be at reach of the Tevatron experiments, depending on the value of a few of the many parameters describing SUSY. We could be seeing such a signal popping out any time in that case, in the tau-pair final state, or in its decay to b-quark pairs, accCDF first, and DZERO later, had tentatively a signal of supersymmetric Higgs in their data, CDF in the tau-pair mode and DZERO in the multi-b-jets mode. Both fizzled out with larger statistics. But one never knows...
12) Observation of Squarks and Gluinos
These things, which are predicted by SUSY models, have been searched extensively by CDF and DZERO since Run I. One looks for events with many jets and a large amount of missing energy, which might originate from the escape of a neutralino. So far, nothing to report. But the large cross section predicted for squarks and gluinos by SUSY theories make a signal quite at reach of the Tevatron experiments, for some values of the parameters.
The list, of course, could go on. Indeed, the about 1000 physicists working for CDF and DZERO are doing their best to cover all the above, plus scores of additional searches for Physics beyond the standard model. It must be noted, however, that despite the coverage of these signals most of the effort is concentrated elsewhere, on a few things that the Tevatron experiments can do better and/or sooner than the Large Hadron Collider. Among them, there are notably three important Physics measurements, which belong to the realm of standard model physics:
1) Exclusion of mass values of the standard model Higgs boson;
2) Measurement of the top quark mass;
3) Measurement of the W boson mass.
The above constitute part of the "core" program of Run II at the Tevatron, and must be pursued with momentum. As far as the Higgs is concerned, it is obvious that the Tevatron wants to say as much as possible on the existence of that particle before the LHC is capable of an overtaking sensitivity; the last two items in the short list, instead, require some explanation.

The reason is that the measurement of the top quark mass is by now dominated by systematic uncertainties (most notably, the jet energy scale), and the systematic uncertainties which the Tevatron faces in the measurements of the top mass are smaller than those that will plague CMS and ATLAS. The size of systematic uncertainties does decrease with the increase of sample sizes -albeit less fast than do statistical errors-, and eventually the LHC is expected to surpass the precision of the Tevatron; but given the amazing job that CDF and DZERO have done, reaching a precision of 0.7% in the top mass value, one expects that it will take many, many years before the best measurement becomes property of CERN.
A similar argument can be made for the W boson mass: here the production rate will not be ten thousand times higher, but "only" a few thousand. Again, the problem comes from systematics, which in this case involve the ultra-precise knowledge of the lepton energy scale, and the understanding of parton distribution functions in a kinematical regime which is much less-well known at the LHC. Again, these factors favor the Tevatron. A precision below 20 MeV on the W boson mass is expected by O(10/fb) measurements of CDF and DZERO, and it is a tough call whether the LHC will ever beat that.
All in all, the Tevatron emerges as a giant in the landscape of particle physics accelerators, thanks to its invaluable scientific output, which has been continuing uninterrupted for over twenty years of operation. I can only hope that the Large Hadron Collider will be able to outdo that, but I believe I will be a retired chessplayer by the time that happens.
Comments