
It is official: the OPERA experiment (above, in a sketch) has found its first tau lepton in one of its bricks (a picture of a brick is shown below). What gives, I am hearing some of you ask. It means that a muon neutrino launched from the CERN laboratories in a 730 km course underground has oscillated into its brother, a tau neutrino, and that the latter has materialized into the charged partner, the tau lepton, inside the OPERA detector.
In other words, the observation spells the direct detection of muon neutrino oscillations into tau neutrinos! This is great news for the whole community of high-energy physics (many dull writers would write "great nu's" I guess).
In case you do not know it, neutrinos are neutral leptons, and together with the charged ones they make up "doublets" just like quarks do: matter is therefore organized into 12 kinds of particles, six quarks and six leptons, and divided into three "families", as shown in the diagram below.
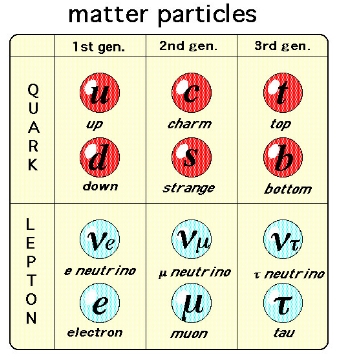
The first family includes the up- and down quarks, the electron, and the electron neutrino; the second family has the charm- and strange quarks, the muon, and the muon neutrino; the third has the top- and bottom quarks, the tau lepton, and the tau neutrino. While only particles from the first family make up ordinary matter, all these 12 particles have similar properties, and their organization looks tidy and pleasant to the eye of a particle physicist.
In the late 1990s the Super-Kamiokande experiment in Japan proved that neutrinos may "oscillate": they may change flavour, such that a muon neutrino may turn into an electron neutrino, or vice-versa. But a muon neutrino had never been directly seen turning into a tau neutrino yet.

When an external detector observes that an interaction has occurred, it extrapolates all observed particles to the brick where the primary neutrino collision has taken place. The brick is extracted from the detector, and its photographic emulsions are developed, allowing the reconstruction software to track the particle trajectories with an accuracy good enough to identify a tau lepton decay, if one has occurred. Tau leptons, in fact, live only few tenths of a trillionth of a second, and in that time they travel only hundreds of microns away from their creation point.
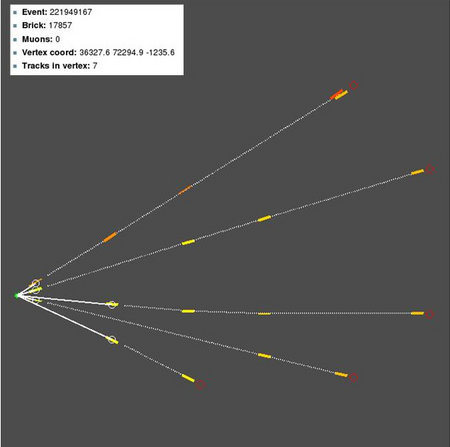
The revolutionary concept will not have escaped an astute reader: OPERA gets dismantled piece by piece during data taking!
There would be a lot to write about the physics of neutrinos and the importance of this research. However, I am rather turned off in my writing vein today by the fact that CERN has issued a very clear and explicative press release. I will for once rely on it parasitically... Here is a clip:
[...]The neutrino puzzle began with a pioneering and ultimately Nobel Prize winning experiment conducted by US scientist Ray Davies beginning in the 1960s. He observed far fewer neutrinos arriving at the Earth from the Sun than solar models predicted: either solar models were wrong, or something was happening to the neutrinos on their way. A possible solution to the puzzle was provided in 1969 by the theorists Bruno Pontecorvo and Vladimir Gribov, who first suggested that chameleon-like oscillatory changes between different types of neutrinos could be responsible for the apparent neutrino deficit.
Several experiments since have observed the disappearance of muon-neutrinos, confirming the oscillation hypothesis, but until now no observations of the appearance of a tau-neutrino in a pure muon-neutrino beam have been observed: this is the first time that the neutrino chameleon has been caught in the act of changing from muon-type to tau-type.
Antonio Ereditato, Spokesperson of the OPERA collaboration described the development as: "an important result which rewards the entire OPERA collaboration for its years of commitment and which confirms that we have made sound experimental choices. We are confident that this first event will be followed by others that will fully demonstrate the appearance of neutrino oscillation".
"The OPERA experiment has reached its first goal: the detection of a tau neutrino obtained from the transformation of a muon neutrino, which occurred during the journey from Geneva to the Gran Sasso Laboratory," added Lucia Votano, Director Gran Sasso laboratories.[...]
The OPERA result follows seven years of preparation and over three years of beam provided by CERN. During that time, billions of billions of muon-neutrinos have been sent from CERN to Gran Sasso, taking just 2.4 milliseconds to make the trip. The rarity of neutrino oscillation, coupled with the fact that neutrinos interact very weakly with matter makes this kind of experiment extremely subtle to conduct. CERN's neutrino beam was first switched on in 2006, and since then researchers on the OPERA experiment have been carefully sifting their data for evidence of the appearance of tau particles, the telltale sign that a muon-neutrino has oscillated into a tau-neutrino. Patience of this kind is a virtue in particle physics research, as INFN President Roberto Petronzio explained:
"This success is due to the tenacity and inventiveness of the physicists of the international community, who designed a particle beam especially for this experiment," said Petronzio. "In this way, the original design of Gran Sasso has been crowned with success. In fact, when constructed, the laboratories were oriented so that they could receive particle beams from CERN".
At CERN, neutrinos are generated from collisions of an accelerated beam of protons with a target. When protons hit the target, particles called pions and kaons are produced. They quickly decay, giving rise to neutrinos. Unlike charged particles, neutrinos are not sensitive to the electromagnetic fields usually used by physicists to change the trajectories of particle beams. Neutrinos can pass through matter without interacting with it; they keep the same direction of motion they have from their birth. Hence, as soon as they are produced, they maintain a straight path, passing through the Earth's crust. For this reason, it is extremely important that from the very beginning the beam points exactly towards the laboratories at Gran Sasso. [...]
While closing a chapter on understanding the nature of neutrinos, the observation of neutrino oscillations is strong evidence for new physics. In the theories that physicists use to explain the behaviour of fundamental particles, which is known as the Standard Model, neutrinos have no mass. For neutrinos to be able to oscillate, however, they must have mass: something must be missing from the Standard Model. Despite its success in describing the particles that make up the visible Universe and their interactions, physicists have long known that there is much the Standard Model does not explain. One possibility is the existence of other, so-far unobserved types of neutrinos that could shed light on Dark Matter, which is believed to make up about a quarter of the Universe's mass.
Comments