The production of resonances decaying to muon pairs is one of the first things one wants to study when a hadron collider starts operation. This is because these particles are extremely well known, so one immediately figures out whether the detector is working properly, what is the resolution on the momenta of the reconstructed particles, etcetera.
Why muon pairs ? Because at a hadron collider muons are well measured and identified with small backgrounds even when they have relatively low momenta. There is a whole family of bodies which decay to muon pairs, which include the J/Psi and Psi(2S) states (bound states of a charm-anticharm quark), the Upsilon 1S,2S,and 3S family (bound states of a bottom-antibottom quark), and of course, the Z boson.
Upsilons are a real treat to see: CMS made a strong effort to analyze as much data as possible before ICHEP, to present a sizable signal of these decays. Look at the mass distribution below: it shows the three resonances standing above small backgrounds (this particular distribution was produced with central muons; CMS produced also a more inclusive distribution, with over three times more Upsilon candidates).

This signal is not qualitatively different from the one obtained with larger statistics by the CDF experiment, which has an excellent resolution for central muons: this tells us that things are in good order. The peaks of the three peaks are exactly where they should be, and their width demonstrates an excellent momentum resolution. Studies performed with this signal together with those of J/Psi mesons and Z bosons already allowed CMS to determine the tracking momentum scale and resolution with great accuracy.
However, with Upsilon mesons things are trickier, and possibly more interesting, than with the other resonances. These particles are indeed well-known, but their production mechanisms at hadron machines is actually still hiding small secrets. In particular, two things require to be stressed.
1) the polarization of Upsilon particles created in the LHC collisions is not known! This actually creates some uncertainty in the number of dimuon decays that can be collected, as a function of the parent body's transverse momentum.
Polarization is a measure of the alignment of the Upsilon spin with its direction of motion. Depending on the particle spin, the two muons get emitted in different angular configurations. This may affect their detection, particularly in a region when the Upsilon has a momentum of about half its rest mass. Have a look at the graph below, which shows the detection efficiency as a function of Upsilon transverse momentum and rapidity.
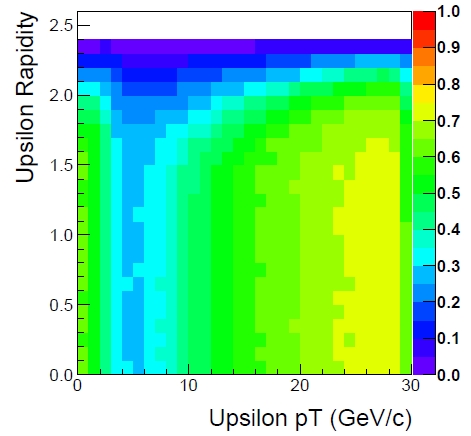
This graph was produced by assuming that the Upsilon has no net polarization (that means that no preferred configuration of the spin is implied). You can see that there is a loss of efficiency for high-rapidity (the upper blue band), where the chance that a muon misses the muon detection system increases; more interesting is to note that there is a whole band of low efficiency for transverse momenta of about 5 GeV.
What is happening is that if the Upsilon is moving at 5 GeV of momentum and it decays with one muon backward-going, the kick from the decay will cancel part of the motion of the system, and the muon will remain with insufficient momentum to reach the detection system (muons with momenta below a few GeV cannot reach the muon chambers of CMS, which are located externally of a strong axial magnetic field and a thick calorimeter).
The "inefficiency band" behaves differently depending on the emission angle of the two muons. So by not knowing the exact production mechanism, and thus not knowing the polarization of the particles, we have uncertainties in the detection efficiency. This will be turned to our advantage once we know our detection efficiency from other sources: we will then be able to determine the polarization by counting these particles as a function of momentum!
2) Upsilon particles are a good means of studying parton distribution functions. This involves comparing their production rapidity with production models which employ different parametrizations of the probability to find partons of given momentum fraction inside the colliding protons.
There are large uncertainties in our knowledge and modeling of the distribution of quarks and gluons of very small momentum inside the proton. These are, however, very important at the LHC. So we need to study light particles going forward! This is because a light particle such as the Upsilon, created in a proton-proton collision in the forward or backward direction, implies that a medium-momentum quark hit a very small-momentum antiquark. By studying the rate of production, we get useful information on the population of these small-momentum partons.
The graph below shows that already with the small statistics collected in the first few months of 7 TeV running of the LHC, we can study the rapidity distribution of Upsilon mesons. This distribution, compared with simulations, will one day allow precise tests of the parton distribution functions.

In summary, it is exciting to see known particles being detected at the LHC, and mined for information that will further our understanding of subnuclear physics. Of course, the LHC is a discovery machine, so eventually the information on the detailed working of the detectors and the production mechanisms for known signals will be turned to the advantage of searches for new physics processes... Stay tuned for these developments!
DISCLAIMER: The opinions expressed in this article are those of the author, and they do not reflect in any way those of the institutions to which he is affiliated. These include the CDF and CMS collaborations, as well as the Italian Institute of Nuclear Physics.
Comments