Can one make plastic from glucose?
When I was young, there appeared on the shelf foods such as kippers that were boil-in-the-bag. I then thought, “is the next stage to eat the bag as well?” This started to look feasible when in the 1980s, ICI had developed bacteria that would convert glucose (or starch, which is a polymer of glucose) into polyhydroxybutyrate (PHB). Given conditions where they cannot convert to glucose all the way into carbon dioxide and water, the bacteria will lay it down into granules of this polymer, in a manner rather similar to that in which Patrick’s yeasts will convert it to ethanol. This polymer is biodegradable, but it didn’t match polypropylene either in properties or price, so it started to fade from the scene. However, in 1992, PHB was produced in transgenic plants, and work has continued (see Plastics You Could Eat – Recycling by Kathy Sykes). Nevertheless, this still has to hit the road, but microbial PHB does survive for use in sutures which can be absorbed by the body, so that the patient doesn’t have to go back to have them removed.
A somewhat more successful polymer which has come on the market in the last ten years is Polylactic acid or polylactide (PLA), made from corn starch, which is also biodegradable and can be made into plates or cups. However, this use of corn may not be ideal, because it takes up land and crops which might otherwise feed hungry humans. Moreover, it has low glass transition between 50 and 80 °C, above which it begins to soften, as in this picture of what happens to a cup that has “held” boiling water.
So, can we make a plastic directly from glucose without using up valuable crop space, or employing pernickety bacteria or GM vegetables to ferment it for us? Until recently, no-one knew how, but recently the door is starting to creak open.
First, catch your Glucose. There’s plenty of it around, in the form of its most abundant polymer cellulose, in trees and grasses. Cows and termites have long employed symbiotic bacteria to do the first stage for them, breaking the cellulose down into glucose (just add water and enzymes.)
The next stage is to gently oxidize the glucose to gluconic acid (which is already found naturally, but there should be plenty of willing micro-organisms around, eager to get a bit of energy for themselves in so doing.) Now as the picture shows, the molecule has hydroxy groups at each end, ready to eliminate water to produce a cyclic molecule called a lactone. (The “lact” bit comes from lactic acid again, which is the “mother of all” molecules that behave in this way.) <?xml:namespace prefix = v ns = "urn:schemas-microsoft-com:vml" /> <?xml:namespace prefix = o ns = "urn:schemas-microsoft-com:office:office" />
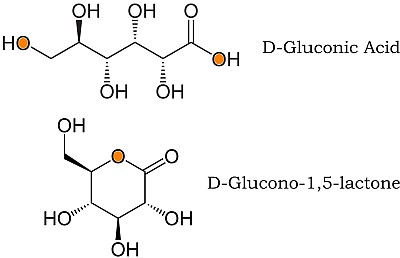
Lactone polymers are already well known, but until recently D-glucono-lactone would not play ball. But last year, Charlotte Williams of Imperial College brought us the glucono polymer illustrated below [1].
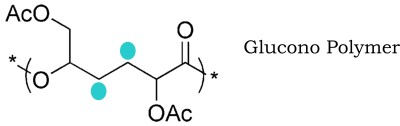
It is made from the gluconolactone in three stages: first, adding the acetyl groups to two of the hydroxyls, reducing the remaining two with hydrogen (blue ellipses showing where the oxygen atoms were), then joining the molecules up like a daisy chain. This in more ways than one, since the favoured chain is actually a big circle of 12 or so individual units.
This first stage polymer still has has one big drawback, and that is its low glass transition of 18°C (remember that polylactide cup?). This would make it more like a goo than "bag" plastic. However, if it could be crosslinked, rather like polyisobutylene to make butyl rubber, would that do the trick? (A lot of cross-linking is what turns your two-pack epoxy resin into a hard adhesive.)
I am told, though, that a way has been found to crosslink this polymer and make a clear film suitable for packaging, which will be published soon. I am watching this space.
[1] Chem. Commun., 2009, 941–943 http://www.rsc.org/Publishing/Journals/CC/article.asp?doi=b817658b
Comments