The fact that I am not a member of DZERO but I still know something about the experiment and its "environment" makes it fun for me to write about their results: despite knowing my way best when I take a walk through CDF or CMS results, I have to be quite careful there to avoid stepping on the toe of some collaborator (and sometimes it feels like their toes cover all the ground), plus I must obey the rules of conduct of my experiments; were all that not sufficient to discourage a blogger, I must avoid at all costs to disclose internal information -that is, ALL except what is published.
With DZERO, on the other hand, I can be more relaxed. Not everybody loves me there, but at least I am not breaking any rule! You don't like what I write about your pet experiment ? Open your own darn blog and start shooting at me from there ;-)
So, what did I find in the arxiv today ? It is a paper describing a new search of flavour-changing neutral current decays of top quark pairs, where about 30,000 of the latter have been produced in something like 300 quadrillion 1.96 TeV proton-antiproton collisions by the Fermilab Tevatron collider. That is a lot of data, and albeit they amount to less than half the total statistics available nowadays in the DZERO data vaults, it is definitely interesting to see what the experiment has to say about rare top quark decays.
FCNC for real dummies
Flavour-changing neutral currents (FCNC) are a chimeric concept in particle physics, and yet their study has been quite fruitful to study in the past. Let me introduce them to you by saying what they are: "currents" are an old-fashioned concept in quantum field theory, and to be accurate I should start discussing destruction and construction operators, which I can't possibly keep at an easy level, since I am no theorist.
So I will be happy to say that a current is a way to describe the propagation of a particle; if this particle can change the flavour of a particle it couples with, it may well be called "flavour-changing". And if the propagating particle is neutral -well, you have it.
The neutral currents we are talking about belong to the class of interactions called "weak interactions" along with the other category, charged currents. Neutral currents are caused by Z bosons, which as you might recall are electrically neutral; charged currents are instead transmitted by W bosons. W and Z bosons are the very heavy elementary particles discovered by Rubbia and collaborators in 1983 at CERN: they are heavy, and can interact weakly but democratically with all matter particles, quarks and leptons.
Now for the "FC" in the acronym: It so happens that W bosons are the only catalysts we know that perform the miracle of transmuting one substance into another: quarks of a certain flavour may become others, lighter (usually) but also heavier (if enough energy is provided!). The same goes with leptons, of course.
The idea of transmuting one substance into another is over two thousand years old: how fantastic would it be to change lead into gold, and how thrilling must have felt to believe it could be done. Fame and impossible riches would be awaiting the discoverer of the recipe! A grand concept, and an ambitious one. Six hundred years after the golden age of alchemists -the middle ages-, we have learned quite a few things, but we now rather keep a lower profile, and content ourselves with trying to figure out whether we do understand everything about the reactions that are capable to change the flavour of a quark or a lepton. Turning lead into gold -well, nowadays it would be more useful to turn CO2 into water.
Back to physics, the reasoning behind the hypothesis of flavour-changing neutral currents goes as follows: W and Z bosons are very close brothers; they belong to a triplet of fields with exactly the same properties in the unbroken electroweak Lagrangian density. Why then can the W play a trick that the Z cannot play ? Well, we do not know, but we have observed since a long time ago that they do not.
The presence of neutral currents capable of changing one quark into another of different flavour (but same colour! Weak bosons do not "see" that property of quarks) would change quite a bit of the phenomenology of elementary particles, especially in particle decays. Some decays of hadrons would be observed with a frequency many orders of magnitude larger than what rare allowed Standard Model reactions sometimes manage to produce; other decays would be reduced in rate to make room for FCNC ones.
We can summarize the picture as follows: all the reactions studied over the course of the last sixty years produce severe limitations on the effective strength that a flavour-changing neutral current may have, because no effect was ever observed that was explainable only with the intervention of FCNCs.
Enter the top quark
Now, there is no real reason to hypothesize that top quarks make an exception. That is, there are reasons, but I do not believe them.... These imply the existence of some particular new physics beyond the Standard Model, which I find not overall too appealing or called for. That is of course only my own gut feeling.
But still, the top quark stimulates us into wondering. The top is forty times heavier than its third-generation partner, the bottom quark; tops live short enough that they do not bind to make hadrons as other quarks do; and top quarks decay almost exclusively into bottom quarks and W bosons -a perfect example of a charged-current weak interaction, by the way. So, could it be that the study of top decays finally discloses to our view the existence of processes that turn top into other quarks, catalyzed by Z bosons ?


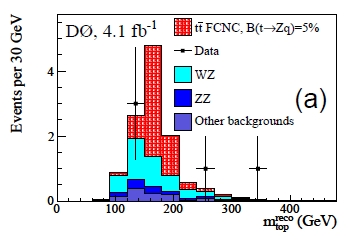

Results of the DZERO search
The data in the graphs above are shown by black points with error bars. It is clear that the data speak in favour of the presence of no FCNC signal: a standard limit-setting procedure then allows to extract an upper limit on the signal in the data. The limit is then translated into a limit on standard "anomalous couplings" which describe the strength of the flavour changing neutral current. This is a rather technical detail, but I only mention it because I wish to show the DZERO result in a summary graph in the plane of these "vector" and "axial-vector" couplings. It is the main result of the paper and it is shown below.

As you can see, this graph has a rather peculiar feature. Despite being a top physics result, the figure includes results of the experiments at the LEP (L3) and the HERA colliders (H1 and ZEUS)! But what do those machines have to do with top physics ? They never produced a single top quark in their lifetime, due to the insufficient energy of their collisions... How could they set limits on FCNCs ?
The fact is that if FCNCs existed, both LEP and HERA could indeed produce top quarks: single top quarks, in fact. LEP II reached a total center-of-mass energy of 209 GeV, which is well enough to produce a top-anticharm pair. A similar process can be produced in the asymmetric collisions of 30 GeV electrons with 920 GeV protons at HERA: just one top quark is produced, and in such cases one is happy enough to observe a top quark to know for sure that it was FCNC processes which produced it. But the experiments of course did not observe top quarks, and that observation was translated again in limits in the vector couplings.
Returning to the DZERO analysis, objectively the added information on these FCNC couplings is not large. However, in the next few years DZERO will be able to analyze the full dataset collected until this summer, and the same will be done by CDF. With their total datasets, the experiments should be able to reach a much tighter limit on these processes, before ATLAS and CMS will become the only players in the game.
Comments