The CMS experiment, I remind you, is one of the four detectors which will start to measure the debris of proton-proton collisions at the Large Hadron Collider, in Geneva, when the latter starts operations a dozen weeks from now. The other three experiments are called Atlas, Alice, and LHCb. Atlas is a direct competitor of CMS in its high-energy discovery program, while Alice focuses on the collisions of heavy ions (which are planned for late 2010), and LHCb mainly studies forward physics in proton-proton collisions, aiming at precision B physics measurements. The poster is shown below, reduced to fit the page; if you click it you may download it in full size powerpoint format (but beware, it is a 8.5 Mb file). The figure on the top right frame in the poster illustrates the arrangement of the experimental facilities along the LHC tunnel under the border of France and Switzerland.

Two words on the plan
CMS will search the Higgs boson from day one, obviously. However, there are a couple of reasons why we do not expect to see it popping up very early on from our data: the reduced center-of-mass energy of the proton-proton collisions, which will only be half of the design 14 TeV for the first 10 months of running; and a somewhat reduced luminosity of the beams in the initial phase, with respect to earlier planning. Both these factors affect the discovery reach of CMS and Atlas.
When the energy of LHC startup was still under debate, eight months ago, CMS quickly produced estimates of the hit on the discovery reach that a reduced energy would take. On that occasion, sensitivity to the Standard Model Higgs boson achievable with 1 inverse femtobarn of collisions at 14 TeV and 10 TeV center-of-mass energy were compared. At the Chamonix meeting in February 2009 this information was debated and digested, and it was understood that it would be useless for discovery purposes to collect just a few inverse picobarns of data in 2009 and then face a long shutdown to perform those repairs which are required for ful-power running. The plan to run continuously through the winter, despite the large increase in electricity bills and the other logistic problems connected with neglecting Christmas holidays, arose back then.
Now that the plan has been finally settled to 7 TeV of c.m. energy, CMS has no revised estimates to show at conferences for the discovery reach at that energy. The 14 TeV case with one inverse femtobarn of data is still interesting, though: it shows the potential of the detector in a "reference" scenario. One can then eyeball that the same results take about four times more data to be obtained at 7 TeV c.m. energy. The assumption of 14 TeV and 1/fb of analyzed data is made in the studies included in the poster and described below.
Where is the Higgs ?
The Standard Model predicts that the Higgs boson is comparatively light. That information is due to its presence in virtual processes which might already have affected the observable value of quantities measured in electron-positron collisions by the LEP and SLC colliders, as well as to the top quark and W boson masses, now precisely measured by the Tevatron experiments.


To show you what I mean when I say that the two bounds have different strength, take then the Gfitter result shown below. The blue curve (or pick the green one if you are only confident in the simpler -2 ln Q method) is the outcome of putting together the result of direct searches with the electroweak indications in a global chisquared. This time, you see that there indeed is a brick wall on the left of 115 GeV -the chisquared literally blows below that value-, while there is just an upward bump in the region 160-170 GeV. Still, combining the direct and indirect information, that mass region is still unlikely by more than three standard deviations (see vertical scale on the right), which means you can bet against it.

All in all, evidence leads us to think that the Standard Model Higgs boson is light - probably between 115 and 140 GeV. In this region the search is difficult, because that particle is then too light to decay frequently to the golden signature of two vector bosons (WW or ZZ), and it needs to be searched in dirtier final states.
But we still look where we can...
A funny story has a drunken looking for his car keys under a street lamp at night. When asked where is he most likely to have lost them, he replies "Oh, I'm pretty sure I dropped 'em about a hundred feet down the road, but it's too dark to search them there". That is more or less what the CMS searches I present in my poster do: they provide information on the sensitivity to a Higgs boson in a range of masses which is disfavoured by indirect and direct searches, as I discussed above.
But things are not so clear-cut, in truth. We will still be able to see a 130 GeV Higgs boson in the H->WW and H->ZZ decay one day, but it might take a while. For advertising purposes, it is better to concentrate where we have a chance of doing better than the Tevatron experiments.
So in my poster I summarize the search for H->WW and H->ZZ events, as well as the search for
H->WW
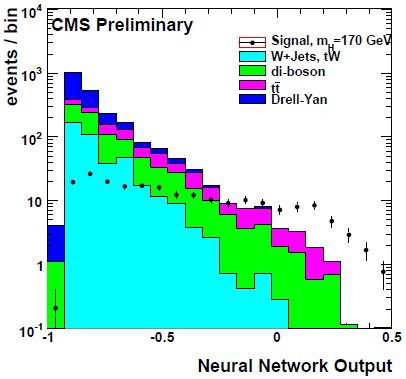

H->ZZ
When the Higgs decays to two Z bosons, the signature is amazingly clean: one may get four high-energy leptons, at least one pair of which makes the mass of the Z (he second pair is of lower effective mass if the Higgs has a mass below 180 GeV, because the corresponding Z boson is then a virtual particle, or as we say "off-mass-shell").

H->tau tau
The third search I present is one for the process called "vector boson fusion", which is another way by which Higgs bosons may be produced at LHC. In vector boson fusion (VBF) diagrams two quarks from the protons spit out W or Z bosons, and it is the latter which fuse together to produce a Higgs. Because of that, the signature includes together with the Higgs two energetic quark jets emitted in forward and backward directions: these are the guys that radiated the vector bosons in the initial part of the reaction.
VBF production is interesting although tenfold more rare than the standard production of Higgs bosons, because the two forward jets effectively provide a means to distinguish the signal. A search for the Higgs decay to a pair of tau leptons, which is hard to distinguish from backgrounds otherwise, can then be carried out.

Conclusions
In conclusion, CMS is a wonderful detector, and the LHC is the most powerful accelerator in the world (that is, will be such in two months), but one which still needs a lot of data to pinpoint rare processes such as Higgs boson production. If the Tevatron folks have been fooled by a downward fluctuation of backgrounds and the Higgs boson is indeed at 160 or 170 GeV, rest assured that CMS will see it with as little as few hundreds inverse picobarns of data; otherwise, we have to realize we are looking forward to a long and painful search in the forthcoming years.
In the next post I will show the other poster I am presenting at PIC 2009, the combination of the H->WW and H->ZZ channels and a comparison of 14 TeV and 10 TeV reaches. Stay tuned!
Comments