Anybody who has had more than a cursory look at quantum mechanics will have come across the correspondence principle, which states that as a system becomes larger it tends towards classical behaviour. Put another way, the quantum numbers in a macroscopic system, say, a ball swinging on a chain, are so large that the energy differences between them are far too small to be measurable. It thus appears that the ball swings in a smooth arc rather than jumping between discrete levels. But how small does this ball, or oscillator, need to be before it starts to exhibit quantum mechanical behaviour? The physicists at the Cleland Group are trying to find out.
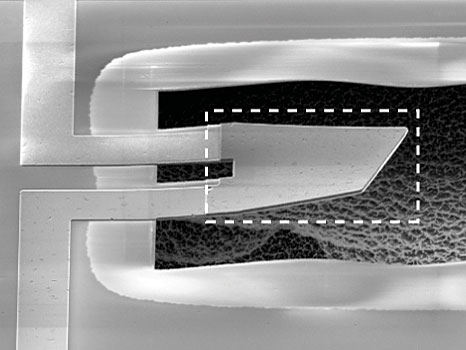
"Researchers at UC Santa Barbara have provided the first clear demonstration that the theory of quantum mechanics applies to the mechanical motion of an object large enough to be seen by the naked eye. Their work satisfies a longstanding goal among physicists.
In a paper published in the March 17 issue of the advance online journal Nature, Aaron O'Connell, a doctoral student in physics, and John Martinis and Andrew Cleland, professors of physics, describe the first demonstration of a mechanical resonator that has been cooled to the quantum ground state, the lowest level of vibration allowed by quantum mechanics. With the mechanical resonator as close as possible to being perfectly still, they added a single quantum of energy to the resonator using a quantum bit (qubit) to produce the excitation. The resonator responded precisely as predicted by the theory of quantum mechanics.
"This is an important validation of quantum theory, as well as a significant step forward for nanomechanics research," said Cleland.
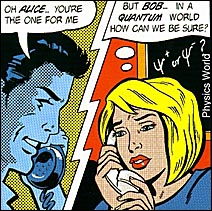
The researchers demonstrated that, once cooled, the mechanical resonator followed the laws of quantum mechanics. They were able to create a single phonon, the quantum of mechanical vibration, which is the smallest unit of vibrational energy, and watch as this quantum of energy exchanged between the mechanical resonator and the qubit. While exchanging this energy, the qubit and resonator become "quantum entangled," such that measuring the qubit forces the mechanical resonator to "choose" the vibrational state in which it should remain.
In a related experiment, they placed the mechanical resonator in a quantum superposition, a state in which it simultaneously had zero and one quantum of excitation. This is the energetic equivalent of an object being in two places at the same time. The researchers showed that the resonator again behaved as expected by quantum theory." [UCSB] You can also hear a brief interview with Andrew Cleland at NPR.
Gives a new meaning to the term quantum mechanical model.
Comments