Whilst it's a gallant attempt to understand the ins and outs of an incredibly complex and poorly understood process, there are a number of misconceptions that I thought would be a good idea to clear up. It's clearly a topic of great interest, because there are over 400 comments on the article.
Before going into these misconceptions, though, let's start with...
A crash course in geomagnetism
Put simply, geomagnetism is a study of the Earth's magnetic field. I guess the guy who kicked this all off was William Gilbert, who, in 1600, made the connection that compasses line up with the Earth as if the Earth was a giant magnet in itself. Previous to this, the concensus was that it was because there was a gigantic magnetic island at the north pole.
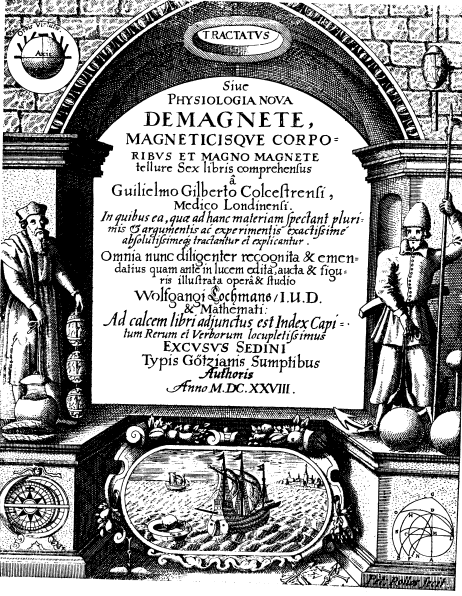
To all intents and purposes, the Earth imagined as a giant magnet is a pretty accurate representation - it really is about 90% dipolar at the surface. And that sort of fitted, because we know that rocks can be magnetic. During the formation of both igneous rocks (those formed from molten rocks) and sedimentary rocks, magnetic minerals can line up in a magnetic field, and the sum total of all these magnetic minerals lining up turns the fluid rock unit into a gigantic magnet. When the temperature of the molten rock cools past a certain temperature (the Curie point) or when the sediments are compacted enough, then these magnetic minerals get frozen in place, and this magnetic field stays in one direction no matter whether the rock is moved into a different field.
So, the rocks of the Earth could form one gigantic magnet. Gilbert demonstrated this using a big spherical magnet, which he called a terella.
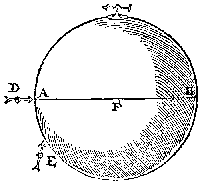
But there was a problem. As soon as 1635, Henry Gellibrand noted evidence that the declination, the difference between true North, as indicated by the pole star, and magnetic North, slowly changed with time. This doesn't work at all with the Earth being a solid magnet. Edmund Halley, in 1692, came up with the radical suggestion that the Earth could be composed of spheres within spheres, each of which had its own magnetic field and moved relative to the other, but ultimately, this isn't physically feasible due to gravity, which would crush such a set-up. Until relatively recently (1950s), one of the leading theories was that magnetism could be an intrinsic property of a rotating object - after all, if spin and magnetic moment were related in electrons and protons, the same could be true for matter at large. But, unfortunately, field strength doesn't seem to weaken with depth in mines, and spinning gold spheres have been shown to not produce any magnetism.
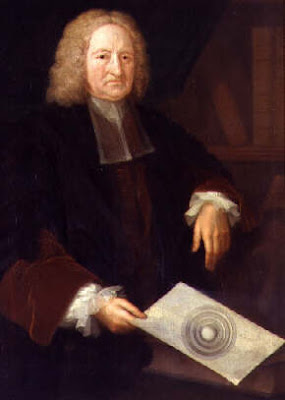
The most accepted theories around today are based on fluid dynamos. We've known for over 100 years from global seismic studies that there is a layer in the Earth inpenetrable to S-waves and so must be liquid. Could this be the region responsible for generating the geomagnetic field?
Building on the observations of inductance by Oersted and Ampere, in the 19th century, Michael Faraday developed the dynamo: a means of generating current by moving a magnet next to a closed circuit. But, he realised, there is another way of inducing a current, and this is by moving the conducting wire relative to the magnet, instead of the magnet relative to the wire. This led to what conventially called a disk dynamo; an admittedly impractical way of making current by spinning a disk of conducting metal next to a magnet - impractical because of the enormous amount of current produced pushed by a low voltage.
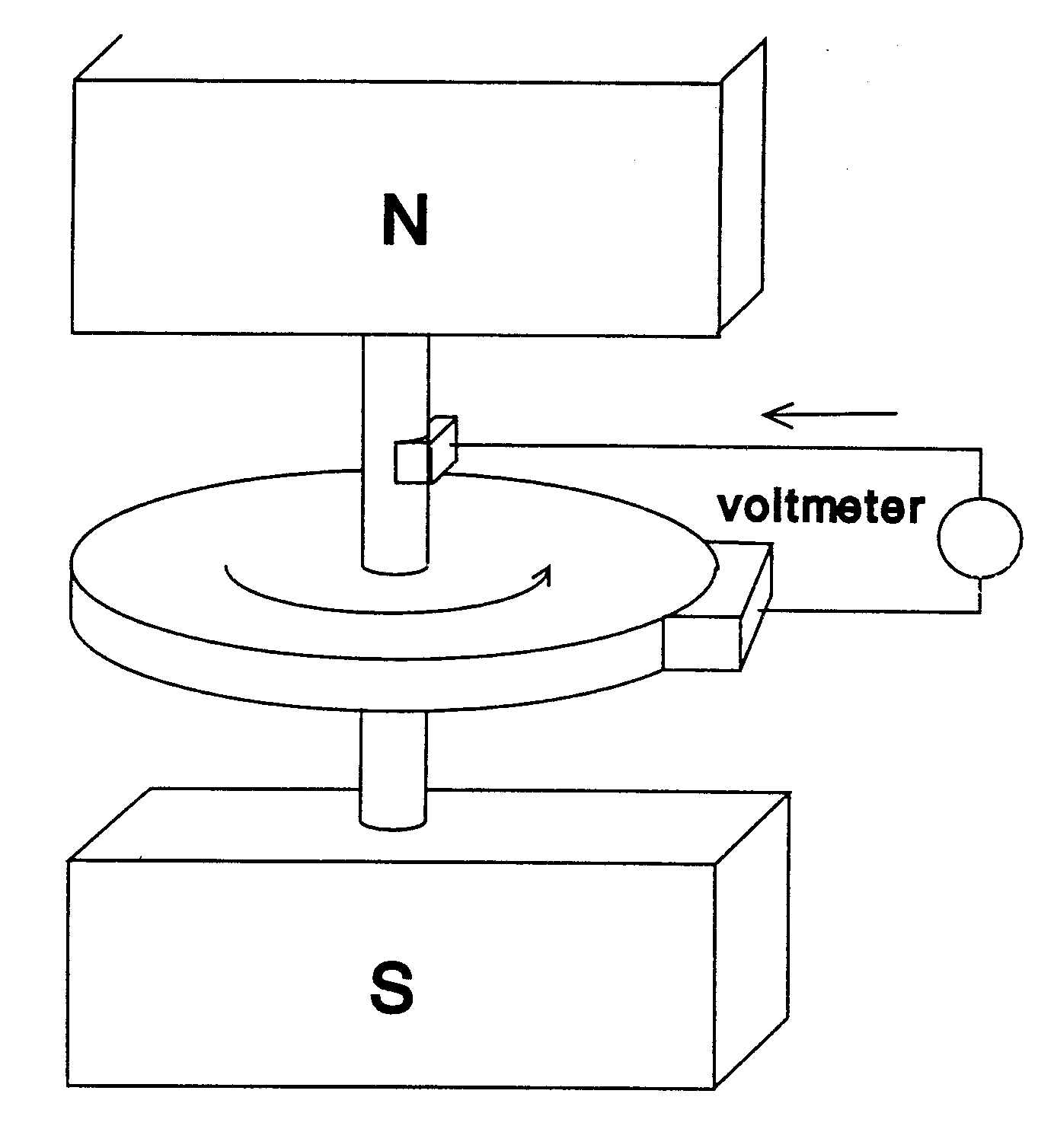
In addition to this, though, he had the insight that the conducting disk needn't be solid, and could instead be a conducting liquid. Faraday actually attempted to measure the induced current in the river Thames by laying a wire across Waterloo bridge*. Its difficult to detect the flow because it is more sparse and less ordered; in normal dynamos we channelize the fields by getting them to flow through wires wrapped in insulators, making them much easier to detect. But the reasoning was sound.
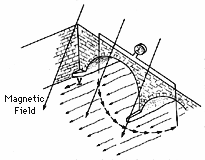
So, in this example, the wire here needs a preexisting magnetic field in order for current to be induced in it, and this induced current in turn will generate its own magnetic field. But could this new magnetic field, in turn, provide the magnetic field for another wire? In other words, could you link two disk dynamos so that each one generates the other's magnetic field?
This is known as a self-exciting dynamo, and whilst in principle it entirely permissible, in practise it is hard to produce, although it may be a matter of scale. On top of that, there is a fundamental difficulty in modeling the Earth's dynamo, because of Thomas Cowling's antidynamo theory, namely that any self-sustaining dynamo in the Earth's core cannot have an axis of symmetry, meaning that the maths is pretty bloody tricky. But any, that's the most accepted theory for how the Earth's geomagnetic field is generated.
Polarity reversals
Polarity reversals are a very important research area for geophysicists, because its essential for understanding how the Earth's geodynamo works, and, of course, to understand the impact on the Earth system in the event of a reversal.
We've tackled this problem from two ends. Firstly, from the paleomag perspective. What can we tell about reversals from the trace that they have left in the sedimentary record? Secondly, we analyse it from numerical models and lab fluid dynamos.
Polarity reversals seem to be an intrinsic feature of these models. Seemingly, in spite of wildly changing any of the parameters by a drastic amount - be it the viscosity, speed of rotation, composition or heat - polarity reversals still occur in the same, spontaneous way. I'll describe our most up to date model of how reversals can occur - remember that these are models and may not necessarily describe precisely what happens in the Earth.
What seems to happen in these models is that in the long term, the geodynamo is pretty happy in its stable dipolar state. That's until, however, during its churning, it stumbles into a less stable multipolar regime. In this new unsteady regime, it is strongly affected by buoyancy forces, unlike during the more rotationally-influenced dipolar regime.
The first stage in the reversal process is a dipole strength reduction, leading to a multi-polar field structure on the core-mantle boundary, which is sometimes quite slow. Next is the multi-polar stage, during which the dipole field sometimes undergoes a precursory reversal and then recovers intensity in its original polarity.
The last stage is the final directional change and intensity recovery in the new polarity. The reversal mechanism initiates with the production and strengthening of reverse magnetic flux in the southern hemisphere of the core. As spots of the reverse flux strengthen and are transported beneath the coreÐmantle boundary, the dipole moment weakens in advance of the actual polarity change, marking the transition from stable dipolar to highly variable multi-polar states. The directional transition is assisted by cross-equatorial meridional circulation.
That's the most recent model, and as you can see, it's a very complex process and is a very active area of research; some of this could very well change within the next few years. The interesting thing is that some of these events seem to be verified by looking at the record that the previous flip left in the geological record.
But there still some misconceptions about the magnetic field that need clearing up.
1. The Strength of the magnetic field is weakening, so we're heading towards a flip
Since the mid 1850s, we have been monitoring the global magnetic field using spherical harmonic analysis, which gives a quantitative description of the Earth's magnetic field in terms of both its direction and strength. It is certainly true that since this time, the strength of the dominant dipole component of the magnetic field has steadily declined by about 5% per century, the decline accelerating somewhat after 1970.
However, we are forgetting that the dipole component is just one component of the geomagnetic field; there are many, more complex, smaller spatial scale components, e.g. the quadrupole and octopole components. Even though measurements of the strength of the magnetic field are decreasing, the geomagnetic field is not getting weaker, only more complex. In fact, these more complex parts are gaining in strength.
It is true that an increase in these complex components is known to be an aspect of reversals. However, the strength of the dipole varies on all sorts of timescales; from centenial to millennial1. The currently observed rate of dipole decay is admittedly quite high, but is still not extraordinarily fast in comparison to the rates of change over the last 7 kyr1. Besides, the present field strength is nearly twice the long-term average field strength2. Indeed, the present field is approximately equal to that during the mid-Cretaceous, when it did not reverse for about 40 million years.
2. We're well overdue a flip

For a start, we have had periods known as superchrons, where the magnetic field didn't flip for over 50 million years. It's only been 780,000 years since the last one. You could argue that we are currently in a "period of increased rate of reversals", but even then, the current chron is barely above average for the Late Cenozoic. The lengths may conform to a Levy distribution, but this doesn't mean that they're not random.
It could be currently flipping. Or it could be on an excursion and not flip for millions of years. Basically, we just can't tell whether we're going to flip or not, and there's no requirement that we do. So, really, it's pointless worrying about it.
3. Because the pole is wandering, we might flip soon
The pole wanders because that's what it does. It drifts westwards, it wanders, and it jerks from time to time. In fact, there are many aborted reversals, when the magnetic poles are observed to move toward the equator for a while, but then move back and align closely with Earth's spin axis.
4. Reversals are linked to extinctions and to large scale biospheric effects
The Earth indeed experiences periods when the surface field strength is puny compared to today, especially during the 5000-10000 year or so transition, and during these periods the Earth's atmosphere (note: not its surface!) is presumably more vulnerable to energetic cosmic particles. The effect of this would most likely result in increased UV-B stress, and increased cloud cover, which would have some effect on the biosphere. And so this is a fair enough question to ask.
The problem is, despite looking closely at very detailed microfossil records, no conclusive link has ever been found3**. Whilst we can't rule out that the biosphere and atmosphere are adversely affected during a reversal, historically, life seems to have got through them OK.
A couple of things, though. As for mammoths, what reversal? There was no reversal 11 Ka when the mammoths died out, and so they were not killed by a geomagnetic reversal. More likely is climate change and man killing them en masse.
I also hope it's clear that flips will not affect plate tectonics or anything on that scale. Why would it? The magnetic field is only strong enough to rotate small magnetic minerals suspended in a liquid, and that is the extent of its strength. On the flip side, it's certainly possible that sinking tectonic plate slabs could sink to the core and affect the circation, but I've never seen any convincing seismic tomography scans that clearly show that slabs can reach that far without being recycled.
Finally, it is certainly possible that large scale astronomical events could cause a reversal - even though, historically, they haven't. But gigantic ion colliders, and other man made activities? If you remember what the magnetic field is being bombarded continually in terms of solar wind, you will realise that man's influence really is puny, particularly on a large scale like this.

5. A self sustaining fluid dynamo is the only way to create a magnetic field
The geodynamo model is our theory for the Earth's geomagnetism, but it needn't be the only way. The fields of Europa and Callisto are produced by induction within Jupiter's magnetic field. And its certainly possible that magnetism can be remnant as well.
In the Sun, the story is a bit simpler, because it is essentially fluid as a whole. I'll describe this in a bit more detail, because its very interesting and demonstrates an important bit of physics. The equatorial region of the Sun rotates more quickly than the poles, which is what drives the dynamo that produces sun spots. Joseph Larmor was the first to apply the idea of a dynamo to the sun's magnetic field, and tentatively suggested that the moving aspect of the dynamo was the convecting plasma. The most major breakthrough after that came with the realisation that, wierdly, magnetic field lines get literally frozen into superconducting plasma particles, and remain aligned even after flow has separated them - or even after they have been catapaulted away from the sun in a flare***. In this way, magnetic field lines are less of a visualization aid and an actual physical construct. They are the direction in a field in which ions and electrons (and their accompanying electric fields and heat) travel most easily; a bit like the grain in a piece of wood that splits easily, and of course can be moved.
Ganymede is an oddity, because has a strong geomagnetic field, and its high degree of central condensation seems to suggest that dynamo action within a metallic core generates the field. But unfortunately, tidal heating is not pervasive enough to melt the core, so our best hypothesis is that it hasn't cooled yet. Or that we don't know enough about generating geomagnetic fields - which is certainly possible.
So, I hope that's cleared up a few things about geomagnetism. As you can see, there's nothing simple about it. However, it's a very active research area, and so, who knows where we will stand in 10 years or so.
----
*He was thwarted from measuring this by small voltages due to chemical processes. Faraday actually speculated that the Gulf Stream could generate a voltage, and if its circuit were closed through the high atmosphere, it could generate there the light of the aurora. Unfortunately, the atmosphere is too poor a conductor of electricity to complete the circuit.
**It has been suggested that there may be a link to the Earth's obliquity, (I can't find a citation) but it's tenuous.
***As the sun rotates, this causes the field lines to become curved round. Solar wind particles on the same field line will therefore be on different meridians than the "roots" of that line on the Sun. The longer these particles have traveled through space, the more have their "roots" rotated and therefore the greater the difference in solar longitude from those roots, suggesting that the magnetic field lines curve to form a spiral, which ultimately become wrapped tighter and tighter.
----
Citations
1. http://onlinelibrary.wiley.com/doi/10.1111/j.1365-246X.2006.03088.x/full
2. http://geology.geoscienceworld.org/cgi/content/abstract/8/12/578
3. http://www.sciencedirect.com/science/article/pii/S0019103508002807
Handy links
http://www.igpp.ucla.edu/people/mkivelson/Publications/ICRUS1572507.pdf
http://www.eolss.net/ebooks/Sample%20Chapters/C01/E6-16-04-01.pdf
http://www.iki.rssi.ru/mirrors/stern/earthmag/mill_5.htm
http://measure.igpp.ucla.edu/solar-terrestrial-luminaries/2000RG000097.pdf
http://www.psc.edu/science/glatzmaier.html
Comments