This question is very much to the point, and its answer is actually not trivial, so I believe it constitutes an excellent topic for an independent post. Mind you, I am sure I wrote more than once in the past about the general topic of reconstructing jet-decaying resonances in hadron collisions, but maybe here we can give the topic a different slant.
Another reason for answering this question in detail is that I believe I am among the expert in this narrow field of research. If you read on, maybe you will agree with this rather presumptuous claim.
The general issue
First of all, let me give you a little background. W and Z electroweak bosons are quite massive particles which mediate the electroweak interaction among quarks and leptons. While in that job the Z is little more than a spectator, the W is a quite busy little worker, since it is responsible for the decay of radioactive nuclei, and for reactions which occur in the core of the stars.
W and Z bosons couple to fermions, and into fermion pairs they decay. Omitting some enlightening but non necessary complications, we can roughly say that they decay two thirds of the time into quark pairs; that is because quarks get a favourable treatment with respect to leptons, since each of them comes in three colours.
Being very massive (they weigh 80.4 and 91.2 GeV respectively, so about 85 and 96 protons each, respectively), W and Z bosons decay imparting high energy to the materialized fermions. Despite the higher rate of quark decays ("hadronic" ones, that's how we call them), it is by detecting their leptonic decays that Rubbia and collaborators discovered the heavy bosons in 1983. To obtain the required energetic collisions Rubbia used protons and antiprotons as projectiles, and the resulting mess created by the collisions did not allow to easily distinguish the two jets of hadrons originated from the materialization of the quarks. On the contrary, the high-energy electrons and muons produced by "leptonic" W or Z decays constituted an excellent, unmistakable signal, which no other process could mimic.
By the mid eighties, W and Z bosons were well-established new particles -the most massive then known yet- and their properties were carefully investigated by the CERN detectors UA1 and UA2 at the SppS collider. Obviously, having observed the decay to leptons, one wanted to verify that the hadronic decays were also occurring, at the rate predicted by electroweak theory: two thirds of the time, roughly speaking.
UA1 and the Dijet Mass Search
There was a problem with this plan. The signature of the decay into quarks of W and Z bosons was too easy to be mimicked by generic quark-antiquark scatterings and annihilations: it featured two energetic jets produced centrally and back-to-back, along with little else. That's a signature that one would call "a dime a dozen", in proton-antiproton collisions! The large background from quantum chromodynamical processes (mediated by strong interactions, and thus "stronger", and more frequent) yielding the same signature made it hard to even collect all the possible W and Z decays at trigger level.
The energy of the two hadronic jets produced in W or Z decay is of about 40-45 GeV -half the boson mass. If the experiments only collected events with at least that energy, they would have then had a very hard time separating the background and the signal using the only very powerful discriminating variable, the resonating dijet invariant mass.
The reason is the following: if you do not apply any selection on jet energies, the dijet mass of a sample of W and Z bosons will make a Gaussian bump at 80 or 90 GeV, while backgrounds will show a featureless, smoothly falling exponential distribution, from the point where the distribution "turns on" -lower values being prevented by the minimum energy at which jets can be reconstructed. However, if you cut away all events which have low-energy jets, you move the "turn-on" rise of the distribution to the right, and you end up with a mass distribution which is peaked too close to the 80-90 GeV where the signal is contributing: the small signal will then be much harder to discern.
To explain better the effect I discussed above, I produced the picture shown below. In red you see the sum of the Gaussian signal of W boson decays to jet pairs (in green) and Z boson decays (in blue). In black, you can see the sum of the signals and an exponentially falling background from strong interaction processes; the background shape has been folded with a turn-on shape, which is the result of imposing a minimum value to the jet transverse energies (transverse energy is what the trigger cuts on). On the top panel, the turn-on has been set by assuming a cut at 12 GeV on jet transverse energies; on the bottom panel, the threshold is at 20 GeV. What a difference a threshold makes! The top figure shows a small but prominent bulge (the background is evidently happy to see us), the bottom one will not excite anybody, and will make you sweat before you can demonstrate it contains the vector boson decay signal!
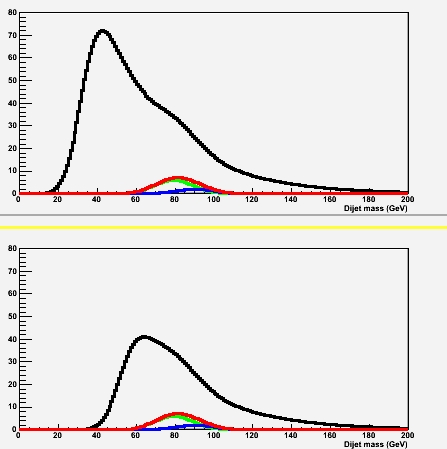
UA1 knew better. They decided to collect with a very low-energy trigger all events with jet pairs, for a significant portion of their data taking. Jet transverse energy thresholds were set at a now impossibly low value of 12 GeV. This produced a large rate of events containing two central, back-to-back jets, and the resulting dijet invariant mass extended down to 40 GeV or so. There was thus plenty of lever arm to understand the true slope of the exponentially falling background from strong interaction processes, and a careful fit allowed the extraction of a tiny "bump" due to the collective signal of W and Z boson decays. The signal can be seen in the figure below, as published in their 1987 article. On the left is the desperately steep dijet mass distribution, on the right the excesses magically extracted by a fit.

Note that W and Z bosons cannot be distinguished well in the UA1 plot (or even in my toy picture): their mass difference is roughly equal to the mass resolution granted by the reconstruction of the jet energies by the UA1 calorimeter, and all one sees is a fat bump, the sum of the two signals. W bosons are more frequently produced than their neutral counterparts because they are lighter and because of the rules of electroweak interactions: there are roughly three W bosons per each produced Z boson.
More recent searches
Now that I have illustrated the first observation of hadronic decays of vector bosons, let me turn to the Tevatron. Would the more powerful machine allow a better, more distinct observation of these decays, and a more detailed study ? Would it be interesting to see them, anyway ?
While the answer to the latter question is "Definitely, yes", because the well-known value of the boson masses would allow, if these particles were reconstructed in jet final states, to precisely calibrate the jet energy measurement (a very complicated and important task in a hadron collider detector, as you can check by reading this discussion of the possible causes of the very recent signal observed by CDF), the answer to the former is not so straightforward.
At a higher collision energy, all processes requiring a significant amount of energy have a larger rate. However, the rise depends on the features of the parton distribution functions (PDF) of the proton. These are probability density functions P(x) that describe how likely it is that a hard proton collision involves a quark or a gluon carrying a fraction x of the total proton momentum (I am simplifying the matter here, but you really do not need to know more about the PDFs).
It turns out that strong interactions producing a dijet system possessing an invariant mass in the 80-90 GeV range grow in rate much more than the vector boson production as one goes from 630 to 1800 GeV of collision energy. So for once, less energy is better! Backgrounds are relatively smaller at the smaller collision energy.
A search for a W/Z decay to jet pairs was actually performed in CDF (but never published), using data collected during the so-called "Run 1c", in 1996: these data were special for CDF because, since a wire of the central tracker had just broken and had required the powering off of a whole CTC sector, it made sense to use all the data acquisition capabilities of the experiment to collect events with jets -that is, events which did not require a working tracker to be reconstructed and understood. And a very low-energy jet trigger was indeed operated during Run 1c, for the purpose of finding these hadronic vector boson decays. Unfortunately, the search never found a signal: indeed, the background was too high, alas.
My Ph.D. Thesis
While some of my colleagues were busy trying to exploit the freshly collected Run 1c data in search of hadronic W and Z decays, I was starting to work at my Ph.D. thesis. Having previously participated in the analysis which had brought to the first observation of all-hadronic top pair decays (that is, events where a top-antitop quark pair was produced, and decayed yielding a total of six hadronic jets, due to the hadronic decay of both W bosons in the chain tt -> WbWb -> jjbjjb), I had some experience in the reconstruction of hadronic decays with jets, and I wanted to do something new.
I soon realized that it would be great if CDF could see the decay of Z bosons to b-quark pairs, a signal which is very similar to that of a low-mass Higgs boson. Searches for the Higgs were still in their infancy in CDF back then, but the issue of reconstructing the Higgs in its jet decay was already hotly debated. But why was I thinking about the Z boson alone (which, at the Tevatron, has a production cross section three times smaller than the W, as already noted) ? And why only the specific decay to b-quark pairs, which happens little less than a sixth of the times ?
The questions have collectively a very simple answer: like the drunkman looking for the watch under the street lamp rather than where he thinks he's lost it, it made sense to look only where something could indeed be found! In fact, while it is hopeless to search for hadronic decays of W and Z bosons altogether, the b-quark jets produced in Z->bb decays have a quite distinctive feature, which allows backgrounds to be reduced by over two orders of magnitude. Strong interactions do produce b-quark jets, but they do so very rarely; therefore, if one requires the jets to contain a signal of the decay of long-lived B particles, one boosts the signal-to-noise ratio by a large factor.
Further, the fact that b-quarks often decay to electrons and muons is crucial: if this were not the case, I would not have had a dataset to start with; instead, I could turn to the so-called "inclusive electron" and "inclusive muon" triggers, which were data collected by requiring the presence of such leptons with moderate cuts on their transverse momentum. Selecting events which had two jets allowed to keep the energy thresholds on these jets as low as needed, partially circumventing the "turn-on" issue I mentioned above.
The b-quark jets thus provided both a feature to collect the events at trigger level (with no "turn-on" bias on the dijet mass distribution), and a significant reduction of backgrounds at analysis level.
It took me some time, but eventually I could put together an optimized kinematic selection, and I was able to extract a veritable signal of Z decays: for the first time at a hadron collider, the Z was observed in its hadronic decay alone. Quite a success, if you ask me. And it gave hope to future searches for the Higgs boson, because the techniques to isolate that signal would later be very similar.

Above you can see the signal I found in inclusive muon data from Run 1 with CDF. The signal is not very strong -it amounts to about 90 events- but it is definitely there. The top inset shows the mass distribution of selected data (red bullets) and background (cyan histogram); the larger picture shows the excess over backgrounds, fit with a simple Gaussian shape. The statistical significance was of "only" three standard deviations, but everybody knew this was a true Z decay signal, since nobody could really doubt that Z bosons ought to be there, at a rate easily computable with simulations. A preprint describing the result is here.
Run 2: a dedicated trigger
While I was finishing my Ph.D. thesis the CDF detector was upgraded, and soon a new data taking started, with the Tevatron sporting a brand new "main injector" boosting its luminosity. And in Run 2, a wonderful new tool became available in CDF: the Silicon Vertex Tracker, a hardware trigger which could measure charged track parameters "on the fly" (literally -we are talking about 20 microseconds), with a precision almost as good as that available offline (Luciano Ristori recently won the Panofsky prize for designing and building the SVT).
By measuring the impact parameter of tracks with 40 micron precision, jets containing bottom quarks could be collected by the trigger system, efficiently and without large backgrounds. The resulting dataset allowed to search for Z bosons with higher statistics, and without some annoying features of the charged leptons which had somehow made things harder in Run 1.
Together with physicists from Harvard and Chicago, Julien Donini (my collaborator for four exciting years in Padova) and I isolated the Z signal in a sample of 600 inverse picobarns of Run 2 data. This time the signal amounted to several thousand events, and it allowed a precise determination of the jet energy scale, the ratio between the energy measured in the data and in the simulation for a jet of given energy.
Let me explain this: by finding the Z signal and fitting its mass in the data and in a simulation of the same process, it was possible to measure the discrepancy of the two values: this is what is called "jet energy scale". We could determine it to be 0.978, with a 0.02 uncertainty. 2%, not bad: this was a nice result, showing that the b-jets, with their peculiarities, were still measured accurately by CDF.
Below you can see the signal we isolated in Run 2. The inset shows the excess over background predictions, while the larger distribution is the dijet mass of event candidates. The smallness of the signal contribution highlights how difficult it is to convince ourselves that the signal is true! The NIM article is available here.

And DZERO ?

In conclusion
I think the above summary is a quick but complete picture of past searches for hadronic decays of vector bosons produced singly in hadron collisions. There would be a lot to say about the W->jj signal that can be isolated in sizable amounts and with negligible backgrounds in top pair decays; or about the diboson signals that have been found in the recent past by CDF. But those are "simpler" searches, because they benefit from the distinctive features of other objects produced in the collision together with the boson.
Much for the same reasons which I tried to explain above when comparing UA1 to CDF, in ATLAS and CMS the search for inclusive hadronic decays of vector bosons is still harder than in CDF and DZERO. I believe somebody crazy enough to search for the hadronic vector boson signal will not be too hard to find -after all these collaborations include over three thousand physicists each, and the rate of nutcases in our community is for sure not smaller than that of any other group of people... So let us keep our fingers crossed: hadronic physics is always entertaining and surprising, at least!
Comments