UPDATE: the live streaming is here.
Below I will give some introductory notions on Higgs physics at the Tevatron; at the bottom of this post I am discussing the actual results.
Introduction
In the 2-TeV proton-antiproton collisions produced at the Tevatron in the course of its 2002-2011 run, Higgs bosons were produced at a considerably reduced rate with respect to the 8-TeV proton-proton collisions at the LHC. The small rate makes the searches of Higgs signals in the most striking decay modes -pairs of Z bosons or pairs of photons- much less effective in America than in Europe. CDF and DZERO, however, have a small advantage: they can rely on a production mechanism called "associated WH or ZH production" which is less background-ridden than it is at the LHC. So I guess that in order to explain well what is going on in this final rush to the Higgs boson, I need to take a step back and explain these production modes first.
Production and Decay
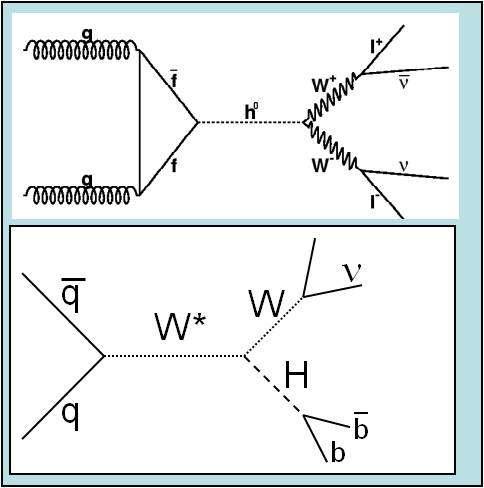
Indeed, as I mentioned the Higgs boson has several different decay modes available when it disintegrates: WW or ZZ pairs, b-quark pairs, tau-lepton pairs, or photon pairs (there are many others, but these are not experimentally interesting at the moment). More on this below.
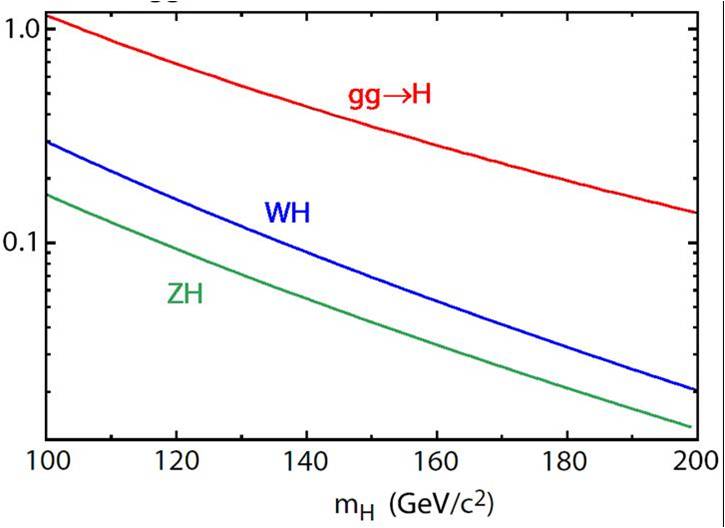
[If your dataset amounts to a luminosity L of ten thousand inverse picobarns and the cross section is 0.1 picobarns, you expect to have bagged a thousand Higgs bosons, since N=σL, where σ is the symbol for the cross section, and L is the luminosity].
You immediately observe that all rates decrease with increasing Higgs mass: that is the result of the increased rarity of quarks and gluons carrying a larger and larger fraction of the proton momentum. The energy they carry in fact is required for the creation of the massive Higgs boson.
So at the Tevatron we are in the ballpark of hundreds of femtobarns for the signal cross section. Making the exercise again to fix the concept in your brain, let us take a 125 GeV Higgs boson and the associated production processes (blue curve plus green curve): you get to calculate that the Tevatron produced N(WH) = 150 fb * 10 / fb = 1500 Higgs bosons in WH production and N(ZH) = 80 fb * 10 /fb = 800 ZH events in the core of each detector.
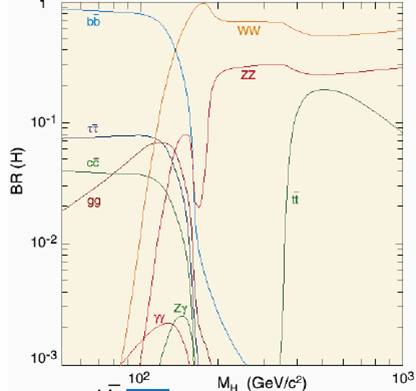
In the end, the particular combination of production mode and decay mode you pick will define a final state which will include different observable particles. This final state is of course mimicked by some processes that have nothing to do with Higgs production: the latter are called "background", and the name of the game is to select the data such that backgrounds are minimized while signals are retained in a sufficient amount to make it observable.
At the Tevatron, the associated production of a Higgs boson and a W or Z boson, with the subsequent decay of the Higgs to a pair of b-quarks, is a promising process because W and Z boson decay signals can be collected cleanly when these particles decay to leptons (electrons or muons), and the signature of a W (or a Z) together with a pair of b-quark jets has backgrounds which are manageable (if barely so).
Note that for a 125 GeV Higgs boson, the decay H->bb is the dominant one, so by concentrating on this channel one is maximizing the number of signal events. On the other hand, the "associated production" mechanism has a rate smaller than the inclusive "gluon fusion" production mode by about a factor of ten (see graph above), so that, too, is a tough choice to make. But perhaps I should not be talking of choices at all here: indeed, the Higgs signal has been sought by CDF and DZERO in virtually all the possible final states -where "possible" should be taken to mean "providing a non-ridiculously-low sensitivity to the signal". Here, however, I am emphasizing the "WH/ZH" production mode and the H->bb decay mode because these are what gives the bulk of the sensitivity of the Tevatron experiments in their combination.
Now I should be telling you why, on the other hand, at the LHC the "Wbb" and "Zbb" final states are not as promising (they have been pursued, but are providing only minor contributions to the overall sensitivity). The LHC, having a much higher centre-of-mass energy, produces b-quark pairs of considerable energy associated to W or Z bosons much more frequently than the Tevatron used to do. So, despite the fact that the production rate of Higgs bosons is higher by one order of magnitude as you increase the centre-of-mass energy fourfold, backgrounds are so large in this final state that the LHC sensitivity is actually worse. Here is one instance where the CDF and DZERO experiments have an advantage, other things being equal: and indeed, other things are equal at this time juncture - I am of course talking of the integrated luminosity which the four experiments can analyze right now: 10 inverse femtobarns of data each.
Enough said about the LHC. So what is the Tevatron showing, after the careful analysis of the full datasets, in search for b-quark pairs associated with a W or Z boson signal ? Well, the title above gave it away already, sort of; but I will wait a few more hours to update this post with the actual results of the experiments... So come back and reload the page, and you will be among the first to know!
Update 1. As a teaser, here is what I can tell you already about the new Tevatron results:
- The Tevatron will present a combination of CDF and DZERO search results
- The updates will be more important for DZERO than CDF: DZERO will update all its results, while CDF will only update their ZH->llbb and VH->vvbb searches (the latter is the signature where you only see two b-jets and there is significant missing energy from the neutrino(s) emitted in the undetected vector boson decay)
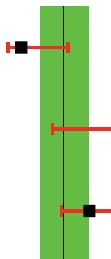
Update 3. Their observed exclusion for a standard model Higgs boson is Mh<103 and 147<Mh<180 GeV. This is looser than their expected exclusion range (which assumes there is NO Higgs boson of course), which is Mh<120 and 139<Mh<184 GeV. The reason is of course traceable to the broad excess that they already presented at winter conferences. Let me remind you, in fact, that the Higgs mass resolution in the Tevatron experiments, which have most of their sensitivity in the H->bb final state, is only of the order of 15 GeV; so a Higgs-related excess of events will degrade the exclusion power in a broad range.
Final update: Okay, now the seminar is on, and I can release the results properly. More information is available at the following links:
- winter 2011 results of Higgs searches
- CDF Higgs results
- DZERO Higgs results
The experiments have made significant progress in the efficiency of b-jet tagging and in the improvement of the mass resolution for pairs of b-quark jets. They both use multivariate discriminants for the selection of the data (Neural Networks and Boosted Decision Trees), and a careful treatment of systematic uncertainties, whose correlation needs to be assessed across the experiments in the combination of results, as well as across the different channels.
The selection is validated in a number of ways. For instance, the standard model production of WW and WZ pairs comes to help. When one W boson decays to leptons and the other boson (W or Z) decays to jet pairs, one gets a final state very similar to the one of WH/ZH production. By selecting the data in search for these signals, one can verify that the observed excesses agree with standard model predictions:
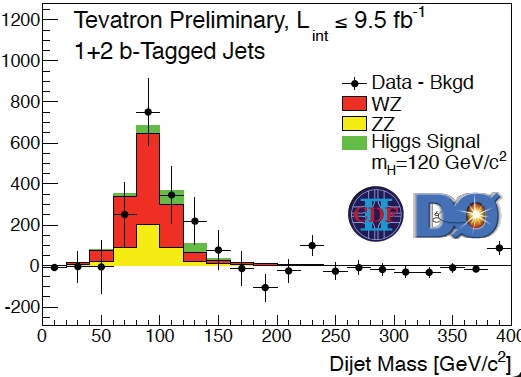
The graph shows a background-subtracted event count as a function of the dijet mass for this diboson selection. Note that here CDF and DZERO data are combined -a rare instance of combination done at histogram level. The black points show the data; the red and yellow histograms show the expected WW and WZ contribution; and the green histogram shows the WH/ZH contribution. Even disregarding the Higgs signal for a moment, one sees that the diboson yield is very well understood in the sample.
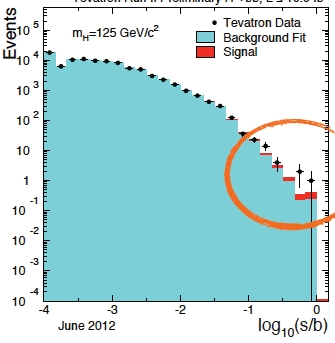
In the H->gamma gamma search the progress has been mostly on the DZERO side. The figure below shows the extracted upper limit on the rate, as always expressed in units of the expected Standard Model prediction. This is obtained by combining CDF and DZERO results for the searches, which are only marginally sensitive to the Higgs signal: you can observe that the observed limit curve (in black) is higher, by about one standard deviation, than the expected limit, in the region of mass where the LHC experiments have indicated the Higgs boson evidence last December.
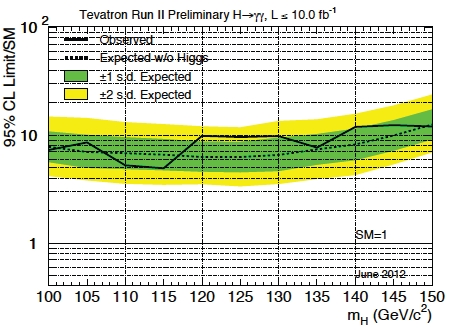
Finally, by running the machinery that extracts limits on the signal rate and p-values for the background-only hypothesis and by combining the H->bb final state results with those of the other searches (H->WW and H->γγ), the Tevatron experiments achieve a nice sensitivity to the Higgs boson. This can be shown in the two figures below.
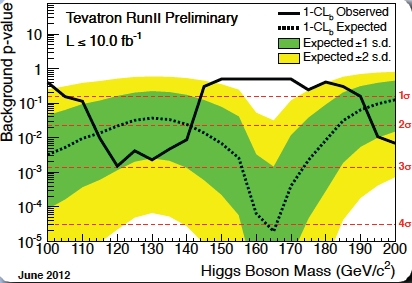
The first one above shows the local p-value of the background-only hypothesis as a function of Higgs boson mass. The black curve shows the local p-value of the combined search, which reaches 3-sigma for a mass of 120 GeV or so; the dashed curve shows the p-value that was predicted if the Higgs boson were actually there (all points of the curve refer to independent hypotheses of Higgs mass). There is compatibility of the result with the expectation, but one notes that the p-value is one-sigma too good. In other words, the Tevatron experiments have been "one-sigma lucky", when their median sensitivity for a 125 GeV Higgs would have been just short of two-sigma.
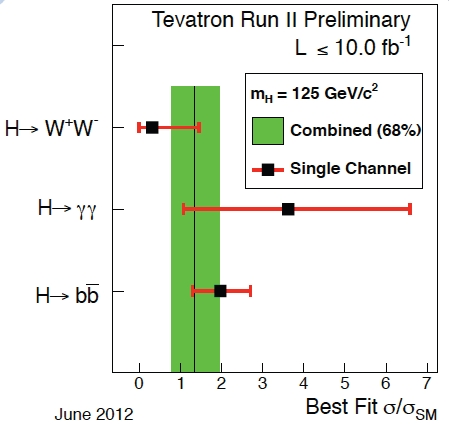
All in all, the signal seen at the Tevatron has a global significance of 2.5 standard deviations. In truth the experiments today are also saying that the highest local significance in the bb final state alone is of 3.2 standard deviations, but this occurs for a Higgs mass hypothesis of 135 GeV, quite far from the true mass of the Higgs boson. True, the experiments do not have the mass resolution of the LHC experiments; but the searches are optimized separately for each mass point, and that is what makes a difference in the p-values of the various searches.
In other words, it is not legal for a correct statistical assessment to pick the highest local significance mass point of the highest-significance channel and then argue that the mass resolution is scarce and thus this local significance is also a global one: indeed, one still has to correct for the look-elsewhere effect - the multitude of masses that have been analyzed. By doing that, the 3.2 sigma become 2.9, and these 2.9 are still an overestimate, because they are derived by ad-hoc picking the most significant channel alone. The correct result is a 2.5 standard deviation effect, and to that the Tevatron should stick in their press releases, in my humble opinion.
In any case, due congratulations to the CDF and DZERO colleagues for this endgame result which, while it will not allow them to eat a part of the discovery cake, does show that the Tevatron was sensitive to the Higgs boson in the end. That is a very nice legacy !
Comments